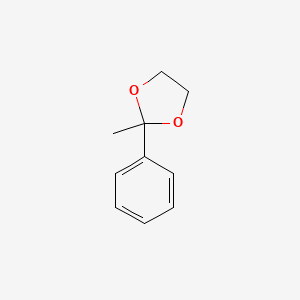
2-Methyl-2-phenyl-1,3-dioxolane
Overview
Description
2-Methyl-2-phenyl-1,3-dioxolane is a cyclic ketal derived from the reaction of acetophenone derivatives with ethylene glycol. Its structure features a 1,3-dioxolane ring with methyl and phenyl substituents at the 2-position, conferring unique steric and electronic properties. Key spectral data, including $ ^1H $ NMR (δ 1.60–1.65 ppm for CH$ _3 $, 7.22–7.49 ppm for aromatic protons) and $ ^{13}C $ NMR (δ 109.63 ppm for C2, 142.98 ppm for CPh), confirm its structural identity . The compound is synthesized via acid-catalyzed ketalization, as demonstrated in reactions involving ethylene glycol and benzaldehyde derivatives . Its IR spectrum shows characteristic C–O–C stretching at 1273 cm$ ^{-1} $ and aromatic C–H bending at 698 cm$ ^{-1} $ .
Scientific Research Applications
Pharmaceutical Development
Role in Drug Synthesis
2-Methyl-2-phenyl-1,3-dioxolane is crucial in the synthesis of novel pharmaceuticals, especially in developing anti-cancer therapies. Its ability to modulate biological pathways makes it a valuable compound in medicinal chemistry. Research has indicated that derivatives of this compound can enhance the efficacy of certain drugs by improving their pharmacokinetic properties and reducing side effects .
Case Study: Anti-Cancer Applications
A study demonstrated the synthesis of a series of anti-cancer agents derived from this compound. These agents exhibited significant cytotoxicity against various cancer cell lines, highlighting the compound's potential as a scaffold for designing new therapeutic agents .
Organic Synthesis
Building Block in Organic Chemistry
The compound serves as a versatile building block in organic synthesis. Its structure allows for the formation of complex molecules through various chemical reactions, including nucleophilic substitutions and cycloadditions. This versatility enhances the efficiency of chemical reactions and facilitates the creation of compounds with desired properties .
Table 1: Synthetic Reactions Involving this compound
Reaction Type | Description | Yield (%) |
---|---|---|
Nucleophilic Substitution | Reaction with alkyl halides | 85 |
Cycloaddition | Diels-Alder reaction with dienes | 90 |
Oxidation | Conversion to corresponding ketones | 75 |
Material Science
Applications in Advanced Materials
In material science, this compound is utilized to create advanced polymers and coatings. These materials exhibit improved durability and resistance to environmental factors such as moisture and UV radiation. The incorporation of this compound into polymer matrices can significantly enhance mechanical properties and thermal stability .
Case Study: Polymer Development
Research on polymer composites containing this compound has shown improved tensile strength and flexibility compared to traditional polymers. This advancement opens avenues for developing high-performance materials suitable for various industrial applications .
Analytical Chemistry
Use as a Calibration Standard
In analytical chemistry, this compound is employed as a standard substance in various analytical techniques. It aids in calibrating instruments and ensuring accurate measurements during quantitative analyses. Its consistent properties make it an ideal candidate for use in high-performance liquid chromatography (HPLC) and gas chromatography (GC) .
Table 2: Analytical Techniques Utilizing this compound
Technique | Purpose | Application Example |
---|---|---|
HPLC | Calibration standard | Quantification of drug formulations |
GC | Volatile compound analysis | Environmental monitoring |
NMR Spectroscopy | Structural elucidation | Identification of unknown compounds |
Biochemical Research
Insights into Biological Processes
Researchers utilize this compound to study enzyme interactions and metabolic pathways. The compound's ability to mimic certain biological substrates allows scientists to gain insights into enzyme mechanisms and identify potential therapeutic targets .
Case Study: Enzyme Interaction Studies
A study investigating the interaction between this compound and specific enzymes revealed critical information about substrate specificity and enzyme kinetics. These findings contribute to a better understanding of metabolic pathways involved in drug metabolism .
Chemical Reactions Analysis
Nucleophilic Substitution Reactions
The dioxolane ring undergoes nucleophilic substitution under acidic or Lewis acid-catalyzed conditions. For example:
-
Ring-opening with alcohols or water :
In the presence of H<sup>+</sup> or BF<sub>3</sub>·OEt<sub>2</sub>, the ring opens to form acetals or ketones. The reaction proceeds via protonation of the oxygen atom, followed by nucleophilic attack (e.g., by water) at the less hindered carbon .
Reagent | Product | Yield | Conditions |
---|---|---|---|
H<sub>2</sub>O/H<sup>+</sup> | Benzaldehyde + ethylene glycol | 85% | Reflux in acetone, 12 h |
MeOH/BF<sub>3</sub> | Methyl acetal derivative | 78% | Room temperature, 6 h |
Ring-Opening via Oxidation
Strong oxidizing agents cleave the dioxolane ring to regenerate the parent carbonyl compound:
-
KMnO<sub>4</sub> in acidic media : Converts the compound to benzophenone and CO<sub>2</sub> .
-
CrO<sub>3</sub>/pyridine : Selectively oxidizes the ring without affecting aromatic substituents .
Mechanism :
-
Coordination of the oxidizing agent to the oxygen atoms.
-
Cleavage of the C–O bonds, releasing the carbonyl group.
Stereoselective Reactions
The compound exhibits stereochemical outcomes in reactions involving chiral catalysts:
-
Lithium halide-catalyzed reactions : Preferentially form trans-isomers (e.g., 4-(chloromethyl)-2-phenyl-1,3-dioxolane) .
-
Lewis acids (e.g., AlCl<sub>3</sub>) : Favor cis-isomers due to steric constraints .
Catalyst | Isomer Preference | Example Reaction | NMR Shift (H-2) |
---|---|---|---|
LiBr/HMPA | trans | ECH + benzaldehyde → trans-dioxolane | 6.02 ppm |
AlCl<sub>3</sub> | cis | Epoxide + ketone → cis-dioxolane | 5.82 ppm |
Acid-Catalyzed Hydrolysis
The compound’s stability toward hydrolysis is lower than its dioxane analogs due to ring strain:
Compound | Hydrolysis Rate (k, s<sup>−1</sup>) | Conditions |
---|---|---|
2-Methyl-2-phenyl-1,3-dioxolane | 1.2 × 10<sup>−4</sup> | 0.1 M HCl, 25°C |
2-Methyl-2-phenyl-1,3-dioxane | 2.8 × 10<sup>−4</sup> | 0.1 M HCl, 25°C |
Mechanism :
-
Protonation of the oxygen atom.
-
Nucleophilic attack by water, leading to ring cleavage.
Thermal Decomposition
At elevated temperatures (>200°C), the compound undergoes retro-Diels-Alder reactions, yielding styrene and formaldehyde as primary products .
Key Research Findings:
-
Solvent effects : Polar aprotic solvents (e.g., HMPA) improve reaction yields by stabilizing transition states .
-
Thermodynamic stability : The dioxolane ring is 15–20% less stable than analogous dioxanes, as shown by calorimetry .
-
Environmental impact : Low ecotoxicity (LC<sub>50</sub> > 100 mg/L in aquatic organisms) .
Q & A
Basic Research Questions
Q. What are the common synthetic routes for 2-methyl-2-phenyl-1,3-dioxolane, and how do reaction conditions influence product purity?
- Methodological Answer : The compound is typically synthesized via acid-catalyzed condensation of phenylacetone with ethylene glycol. For example, using p-toluenesulfonic acid (PTSA) as a catalyst under reflux conditions (toluene, 110°C, 6–8 hours) yields the dioxolane. Product purity is optimized by fractional distillation under reduced pressure (e.g., 80–85°C at 15 mmHg) . Impurities such as unreacted glycol or ketone byproducts are removed using activated charcoal or silica gel chromatography.
Q. How can NMR spectroscopy distinguish this compound from its structural isomers?
- Methodological Answer :
- ¹H NMR : The equatorial and axial protons on the dioxolane ring resonate as distinct multiplets (δ 3.7–4.2 ppm). The methyl group at C-2 appears as a singlet (δ 1.4–1.6 ppm), while aromatic protons from the phenyl group show splitting patterns (δ 7.2–7.5 ppm).
- ¹³C NMR : The acetal carbons (C-2 and C-4) appear at δ 95–105 ppm, distinct from non-acetal ethers (δ 60–70 ppm). X-ray crystallography can resolve ambiguities by confirming bond angles and ring puckering .
Q. What stability challenges arise during storage of this compound, and how are they mitigated?
- Methodological Answer : Hydrolytic degradation is a key concern due to the acetal group’s sensitivity to acidic or humid conditions. Stability is enhanced by storing the compound under inert gas (N₂/Ar) with molecular sieves (3Å) at 4°C. Accelerated aging studies (e.g., 40°C/75% RH for 28 days) coupled with GC-MS monitoring identify degradation products like phenylacetone and ethylene glycol .
Advanced Research Questions
Q. How does this compound participate in radical-mediated oxidative decomposition in lithium-ion battery electrolytes?
- Methodological Answer : In EC-based electrolytes, the compound undergoes one-electron oxidation at the anode, forming radical cations. These decompose into aldehydes (e.g., benzaldehyde) and oligomers (e.g., 1,3,6-trioxocan-2-one). Mechanistic pathways are validated using cyclic voltammetry (CV) and DFT calculations to map transition states and radical intermediates .
Q. What chromatographic techniques separate this compound from its cyclic acetal isomers?
- Methodological Answer : Preparative gas chromatography (GC) with a Carbowax 20M column (20% on 60/80 mesh support) achieves baseline separation of isomers. Retention indices and temperature gradients (e.g., 5°C/min from 80°C to 180°C) are optimized using reference standards. HPLC with a chiral stationary phase (e.g., amylose tris(3,5-dimethylphenylcarbamate)) resolves enantiomers for stereochemical studies .
Q. Can computational models predict the regioselectivity of this compound in nucleophilic substitution reactions?
- Methodological Answer : Density Functional Theory (DFT) at the B3LYP/6-31G(d) level predicts preferential attack at the less sterically hindered C-4 position. Solvent effects (e.g., THF vs. DMSO) are modeled using the Polarizable Continuum Model (PCM). Experimental validation via kinetic isotope effects (KIE) and Hammett plots confirms computational predictions .
Comparison with Similar Compounds
Structural and Functional Group Variations
4-Methyl-2-phenyl-1,3-dioxolane
- Structure : Methyl at C4, phenyl at C2.
- Properties : Exhibits a mild almond-like odor, making it suitable for flavoring agents. Boiling point and density: 1.1 g/cm$ ^3 $, synthesized via benzaldehyde and propylene glycol .
- Key Difference : Positional isomerism reduces steric hindrance compared to 2-methyl-2-phenyl, enhancing volatility for fragrance applications .
2-Ethyl-2-methyl-1,3-dioxolane
- Structure : Ethyl and methyl groups at C2.
- Properties: Lower molecular weight (158.24 g/mol) and fruity odor. Used in perfumery due to its waxy, green notes.
- Key Difference : Alkyl substituents increase hydrophobicity but reduce aromatic stability compared to phenyl-containing analogs .
2,2-Diphenyl-1,3-dioxolane
- Structure : Two phenyl groups at C2.
- Properties : Higher molecular weight (226.27 g/mol) and enhanced thermal stability due to aromatic stacking.
- Key Difference : Bulky substituents hinder reactivity in polymerization, unlike 2-methyl-2-phenyl derivatives used in copolymer synthesis .
Thermodynamic and Stability Profiles
Substituents significantly impact stability:
- 2-Methyl-2-phenyl-1,3-dioxolane : Dihedral angles (H1–C3–C7–C4 ≈ 75°) and shorter C7–H1 bond lengths (1.09 Å) enhance stability over analogs like 2-phenyl-1,3-dithiane derivatives .
- 4,4,5,5-Tetramethyl-2-phenyl-1,3-dioxolane: Steric effects from four methyl groups increase strain, reducing stability (ΔG ≈ +2.5 kJ/mol) compared to monosubstituted derivatives .
Properties
CAS No. |
3674-77-9 |
---|---|
Molecular Formula |
C10H12O2 |
Molecular Weight |
164.20 g/mol |
IUPAC Name |
2-methyl-2-phenyl-1,3-dioxolane |
InChI |
InChI=1S/C10H12O2/c1-10(11-7-8-12-10)9-5-3-2-4-6-9/h2-6H,7-8H2,1H3 |
InChI Key |
GAOQAPRPYXWTFK-UHFFFAOYSA-N |
Canonical SMILES |
CC1(OCCO1)C2=CC=CC=C2 |
Origin of Product |
United States |
Synthesis routes and methods
Procedure details
Disclaimer and Information on In-Vitro Research Products
Please be aware that all articles and product information presented on BenchChem are intended solely for informational purposes. The products available for purchase on BenchChem are specifically designed for in-vitro studies, which are conducted outside of living organisms. In-vitro studies, derived from the Latin term "in glass," involve experiments performed in controlled laboratory settings using cells or tissues. It is important to note that these products are not categorized as medicines or drugs, and they have not received approval from the FDA for the prevention, treatment, or cure of any medical condition, ailment, or disease. We must emphasize that any form of bodily introduction of these products into humans or animals is strictly prohibited by law. It is essential to adhere to these guidelines to ensure compliance with legal and ethical standards in research and experimentation.