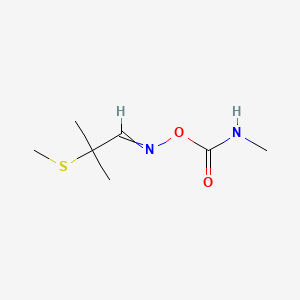
Temik
Overview
Description
Temik is a chemical compound known for its use as an insecticide, nematicide, and acaricide. It is commonly referred to by its trade name, this compound. This compound is widely used in agriculture to control a variety of pests that affect economically important crops.
Preparation Methods
Synthetic Routes and Reaction Conditions
The synthesis of Temik involves several steps. The starting material is typically 2-methyl-2-(methylthio)propanal, which undergoes a reaction with hydroxylamine to form the oxime derivative. This intermediate is then reacted with methyl isocyanate to produce the final compound.
Industrial Production Methods
Industrial production of this compound follows a similar synthetic route but on a larger scale. The reaction conditions are optimized to ensure high yield and purity. The process involves careful control of temperature, pressure, and the use of catalysts to facilitate the reactions.
Chemical Reactions Analysis
Types of Reactions
Temik undergoes several types of chemical reactions, including:
Oxidation: The compound can be oxidized to form sulfoxides and sulfones.
Reduction: Reduction reactions can convert the oxime group back to the corresponding amine.
Substitution: The compound can undergo nucleophilic substitution reactions, particularly at the oxime and carbamoyl groups.
Common Reagents and Conditions
Oxidation: Common oxidizing agents include hydrogen peroxide and potassium permanganate.
Reduction: Reducing agents such as lithium aluminum hydride or sodium borohydride are used.
Substitution: Nucleophiles like amines or thiols can be used under mild conditions.
Major Products Formed
Oxidation: Sulfoxides and sulfones.
Reduction: Corresponding amines.
Substitution: Various substituted derivatives depending on the nucleophile used.
Scientific Research Applications
Temik has several scientific research applications:
Chemistry: Used as a model compound to study reaction mechanisms and kinetics.
Biology: Investigated for its effects on various biological systems, particularly its role as an acetylcholinesterase inhibitor.
Medicine: Explored for potential therapeutic applications due to its bioactivity.
Industry: Widely used in agriculture to control pests, improving crop yield and quality.
Mechanism of Action
The primary mechanism of action of Temik is through inhibition of acetylcholinesterase, an enzyme crucial for nerve function. By inhibiting this enzyme, the compound disrupts the normal transmission of nerve impulses, leading to paralysis and death of the pests. The molecular targets include the active site of acetylcholinesterase, where the compound binds and prevents the breakdown of acetylcholine.
Comparison with Similar Compounds
Similar Compounds
Carbofuran: Another carbamate insecticide with a similar mechanism of action.
Methomyl: A carbamate insecticide used for similar purposes.
Aldicarb-sulfoxide: An oxidized derivative of aldicarb with similar properties.
Uniqueness
Temik is unique due to its high potency and effectiveness against a wide range of pests. Its ability to be absorbed by plants and act systemically makes it particularly valuable in agricultural applications.
Properties
The carbamate insecticides are reversible cholinesterase-inhibitors. They cause this effect by reversible carbamylation of the enzyme acetylcholinesterase, allowing accum of acetylcholine, as with the organophosphate insecticides. ... While the organophosphate insecticides cause irreversible inhibition of the cholinesterase enzymes, the carbamyl-enzyme complex is reversible & dissociates far more readily than the organophosphate complex. The clinical syndrome is more benign & of much shorter duration with the carbamate insecticides. Unlike the the organophosphates, the carbamates poorly penetrate the CNS. /Carbamate insecticides/ | |
Molecular Formula |
C7H14N2O2S C7H14N2O2S CH3SC(CH3)2CH=NOCONHCH3 |
Molecular Weight |
190.27 g/mol |
IUPAC Name |
[(2-methyl-2-methylsulfanylpropylidene)amino] N-methylcarbamate |
InChI |
InChI=1S/C7H14N2O2S/c1-7(2,12-4)5-9-11-6(10)8-3/h5H,1-4H3,(H,8,10) |
InChI Key |
QGLZXHRNAYXIBU-UHFFFAOYSA-N |
impurities |
Impurities likely to be present in technical aldicarb in concentrations of over 100 ppm include the following: (CH3)2C(SCH3)CH:NOH, (CH3)2C(OC2H5)CH:NOCONHCH3, (CH3)2C(SOCH3)CH:NOCONHCH3, (CH3)2C(SCH3)CN, CH3NHCONHCH3, CH3NHCONC(CONHCH3)CH3, (CH3)2C(SCH3)CH:NOCON(CONHCH3)CH3 |
SMILES |
CC(C)(C=NOC(=O)NC)SC |
Canonical SMILES |
CC(C)(C=NOC(=O)NC)SC |
boiling_point |
Decomposes (NTP, 1992) decomposes |
Color/Form |
Crystals from isopropyl ether |
density |
1.195 at 77 °F (EPA, 1998) Specific gravity: 1.1950 at 25 °C 1.195 |
melting_point |
210 to 214 °F (EPA, 1998) 99-100 °C 100 °C 210-214 °F |
physical_description |
Aldicarb appears as white crystals with a slightly sulfurous odor. Commercial formulations are granular Used as an insecticide, acaricide, and nematocide. (EPA, 1998) White crystals with a slightly sulfurous odor; [CAMEO] COLOURLESS CRYSTALS. White crystals with a slightly sulfurous odor. Commercial formulations are granular. |
solubility |
0.1 to 1.0 mg/mL at 72 °F (NTP, 1992) Sparingly soluble in certain organic solvents, most soluble in chloroform and acetone Aldicarb solubilities (expressed as %) at various temperatures. Table: Percent Solubility [Table#3193] 350 g/kg, acetone; 300 g/kg, dichloromethane; 150 g/kg, benzene; 150 g/kg, xylene; all at 25 °C In water, 4,930 mg/L at 20 °C Practically insoluble in hexane Solubility in water, g/100ml at 25 °C: 0.6 |
vapor_pressure |
Less than 0.5 at 68F (EPA, 1998) 0.0000347 [mmHg] 2.9X10-5 mm Hg at 20 °C Vapor pressure, Pa at 25 °C: 0.01 <0.5 mmHg |
Origin of Product |
United States |
Synthesis routes and methods I
Procedure details
Synthesis routes and methods II
Procedure details
Synthesis routes and methods III
Procedure details
Synthesis routes and methods IV
Procedure details
Retrosynthesis Analysis
AI-Powered Synthesis Planning: Our tool employs the Template_relevance Pistachio, Template_relevance Bkms_metabolic, Template_relevance Pistachio_ringbreaker, Template_relevance Reaxys, Template_relevance Reaxys_biocatalysis model, leveraging a vast database of chemical reactions to predict feasible synthetic routes.
One-Step Synthesis Focus: Specifically designed for one-step synthesis, it provides concise and direct routes for your target compounds, streamlining the synthesis process.
Accurate Predictions: Utilizing the extensive PISTACHIO, BKMS_METABOLIC, PISTACHIO_RINGBREAKER, REAXYS, REAXYS_BIOCATALYSIS database, our tool offers high-accuracy predictions, reflecting the latest in chemical research and data.
Strategy Settings
Precursor scoring | Relevance Heuristic |
---|---|
Min. plausibility | 0.01 |
Model | Template_relevance |
Template Set | Pistachio/Bkms_metabolic/Pistachio_ringbreaker/Reaxys/Reaxys_biocatalysis |
Top-N result to add to graph | 6 |
Feasible Synthetic Routes
Disclaimer and Information on In-Vitro Research Products
Please be aware that all articles and product information presented on BenchChem are intended solely for informational purposes. The products available for purchase on BenchChem are specifically designed for in-vitro studies, which are conducted outside of living organisms. In-vitro studies, derived from the Latin term "in glass," involve experiments performed in controlled laboratory settings using cells or tissues. It is important to note that these products are not categorized as medicines or drugs, and they have not received approval from the FDA for the prevention, treatment, or cure of any medical condition, ailment, or disease. We must emphasize that any form of bodily introduction of these products into humans or animals is strictly prohibited by law. It is essential to adhere to these guidelines to ensure compliance with legal and ethical standards in research and experimentation.