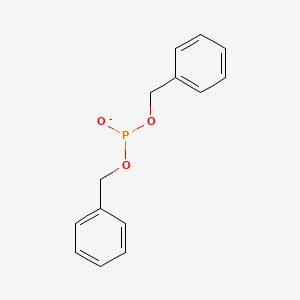
Dibenzyl phosphite
- Click on QUICK INQUIRY to receive a quote from our team of experts.
- With the quality product at a COMPETITIVE price, you can focus more on your research.
Overview
Description
Dibenzyl phosphite is an organophosphorus compound with the chemical formula C14H15O3P. It is a phosphite ester, characterized by the presence of two phenylmethyl groups attached to a phosphorous atom. This compound is known for its applications in various fields, including chemistry, biology, and industry.
Preparation Methods
Synthetic Routes and Reaction Conditions
Dibenzyl phosphite can be synthesized through several methods. One common approach involves the reaction of phenylmethyl alcohol with phosphorus trichloride in the presence of a base such as pyridine. The reaction typically proceeds as follows:
3C6H5CH2OH+PCl3→(C6H5CH2O)3P+3HCl
The reaction is carried out under anhydrous conditions to prevent hydrolysis of the phosphorus trichloride. The product, bis(phenylmethyl) phosphite, is then purified through distillation or recrystallization.
Industrial Production Methods
In industrial settings, bis(phenylmethyl) phosphite is produced on a larger scale using similar synthetic routes. The process involves the careful control of reaction conditions, such as temperature and pressure, to optimize yield and purity. Industrial production may also involve the use of continuous flow reactors to enhance efficiency and scalability.
Chemical Reactions Analysis
Phosphorylation via the Atherton-Todd Reaction
DBP participates in the Atherton-Todd reaction, a classical method for synthesizing phosphate esters. This reaction involves the activation of DBP with carbon tetrachloride (CCl₄) or bromotrichloromethane (CBrCl₃) in the presence of a base, leading to the formation of reactive intermediates like dibenzyl chlorophosphate.
Mechanism:
-
Deprotonation of DBP by a base (e.g., triethylamine or NaOH) generates a phosphite anion.
-
Nucleophilic attack on CCl₄ or CBrCl₃ forms a chlorophosphate intermediate.
-
Reaction with amines or alcohols yields N-phosphorylated amines or phosphate esters .
Reaction Conditions | Catalyst/Reagent | Product | Yield |
---|---|---|---|
CCl₄, triethylamine, 0–5°C | DMAP | Dibenzyl phenyl phosphate | 69% |
CBrCl₃, KOH | – | Tetrabenzyl pyrophosphate | 95% |
Key Findings :
-
Bromotrichloromethane accelerates the reaction due to easier nucleophilic displacement of bromine vs. chlorine .
-
The reaction is sensitive to moisture and air, requiring anhydrous conditions .
Hydroxylation with Permanganate
DBP undergoes hydroxylation in alkaline media using potassium permanganate (KMnO₄) to produce dibenzyl phosphate. This method avoids harsh reagents like PCl₃ and improves purity .
Reaction Scheme:
(C₆H₅CH₂O)₂P(O)H+KMnO₄NaOH(C₆H₅CH₂O)₂P(O)OH
Parameter | Value |
---|---|
Temperature | 60–80°C |
Reaction Time | 4–6 hours |
Yield (crude) | 95% |
Purity after refining | 99.5% (ethyl acetate/petroleum ether) |
Applications :
-
The product, dibenzyl phosphate, is a precursor for hydrophobic probes targeting prostate-specific membrane antigen .
Transesterification Reactions
DBP exhibits high reactivity in transesterification with alcohols, facilitated by microwave (MW) irradiation or continuous flow systems. This two-step process forms mixed phosphite intermediates.
Example with n-Butanol:
-
Step 1 : DBP reacts with n-butanol to form monobenzyl butyl phosphite.
Condition | Batch (MW) | Continuous Flow |
---|---|---|
Temperature | 180°C | 225°C |
Time/Flow Rate | 40 min | 0.15 mL/min |
Conversion | 84% | 94% |
Yield | 73% | 85% |
Advantages :
Selective Phosphorylation of Primary Alcohols
DBP enables regioselective phosphorylation of primary alcohols in unprotected polyols using a catalytic system of iodine and pyridine.
Mechanism:
-
Iodine oxidizes DBP to iododibenzyl phosphate.
-
Pyridine forms a reactive pyridinium intermediate.
-
Selective attack on primary alcohols occurs due to steric and electronic factors .
Substrate | Product | Selectivity |
---|---|---|
α-Diols | Primary phosphate esters | >90% |
Glycerol | 1-Phosphoglycerol | 85% |
Applications :
Reactivity in Protic Solvents
DBP hydrolyzes in water to form phosphoric acid derivatives, limiting its use in aqueous environments.
Solubility | Reactivity |
---|---|
DMSO, Methanol | Stable |
Water | Rapid hydrolysis |
Scientific Research Applications
Applications in Organic Synthesis
1. Atherton–Todd Reaction
Dibenzyl phosphite plays a significant role in the Atherton–Todd reaction, which is used to synthesize phosphoramidates. This reaction involves the interaction of dialkyl phosphites with primary amines under specific conditions. The products formed can serve as intermediates in various chemical transformations, including the synthesis of biologically active compounds and flame retardants .
2. Prodrug Development
This compound has been investigated for its potential as a prodrug in medicinal chemistry. For example, dibenzyl (methoxycarbonyl)phosphonate was prepared and studied for its hydrolysis kinetics. The rapid hydrolysis of this compound suggests that this compound derivatives could be designed to release active pharmaceutical ingredients in a controlled manner .
Case Study 1: Synthesis of Phosphoramidates
In a study examining the reactions between azido alcohols and dibenzyl phosphoramidites, it was found that stable azido phosphite intermediates could be formed effectively at room temperature. This highlights the utility of this compound in synthesizing complex organic structures under mild conditions .
Case Study 2: Flame Retardant Applications
Research has shown that this compound can be utilized in developing flame retardant materials. The modification of phenolic compounds with this compound enhances their thermal stability and resistance to combustion, making it valuable in materials science for safety applications .
Table 1: Comparison of Synthesis Methods for this compound
Method | Reactants | Conditions | Yield (%) |
---|---|---|---|
Reaction with PCl3 | Benzyl alcohol + PCl3 | Room temperature | 85 |
Hydrolysis of Dibenzyl Chloride | Dibenzyl chlorophosphate + H2O | Reflux | 90 |
Reaction with Phosphorus Oxychloride | Benzyl alcohol + POC13 | Room temperature | 80 |
Table 2: Applications of this compound
Application Area | Description |
---|---|
Organic Synthesis | Used as a reagent in the Atherton–Todd reaction for synthesizing phosphoramidates |
Medicinal Chemistry | Investigated as a prodrug for controlled release of active compounds |
Material Science | Incorporated into flame retardant formulations to enhance thermal stability |
Mechanism of Action
The mechanism of action of bis(phenylmethyl) phosphite involves its ability to donate or accept electrons, making it a versatile reagent in various chemical reactions. In biological systems, it can interact with enzymes and other proteins, potentially inhibiting their activity by forming stable complexes. The molecular targets and pathways involved depend on the specific application and the nature of the interacting molecules.
Comparison with Similar Compounds
Similar Compounds
Triphenyl phosphite: Another phosphite ester with three phenyl groups attached to phosphorus.
Dimethyl phenyl phosphite: A phosphite ester with two methyl groups and one phenyl group attached to phosphorus.
Diethyl phenyl phosphite: A phosphite ester with two ethyl groups and one phenyl group attached to phosphorus.
Uniqueness
Dibenzyl phosphite is unique due to the presence of two phenylmethyl groups, which impart specific chemical properties and reactivity. Compared to other phosphite esters, it offers distinct advantages in terms of stability and versatility in chemical reactions.
Q & A
Basic Research Questions
Q. What are the optimal conditions for synthesizing dibenzyl phosphite, and how can yield be maximized?
this compound is synthesized via transesterification using triethyl phosphite, benzyl alcohol, and pentaerythritol with an organic tin catalyst. Optimal conditions include a reaction temperature of 130–140°C, a 2-hour reaction time, and a 1:2:2 molar ratio of reactants, achieving yields up to 95% . For lab-scale synthesis, ensure inert atmosphere conditions and rigorous purification via vacuum distillation to minimize side reactions.
Q. How is the molecular structure of this compound validated, and what analytical techniques are recommended?
Key structural features include a phosphorus center bonded to two benzyloxy groups and a hydroxyl group. Use nuclear magnetic resonance (NMR) spectroscopy (³¹P and ¹H) to confirm the presence of characteristic peaks (e.g., ³¹P NMR: δ ~10–12 ppm for P–O bonds). Mass spectrometry (MS) and infrared (IR) spectroscopy can corroborate molecular weight (262.24 g/mol) and functional groups (P–O stretching at ~980 cm⁻¹) .
Q. What are the primary applications of this compound in organic synthesis?
It is widely used for:
- Phosphorylation of phenols : React with phenols in acetonitrile using N-ethyldiisopropylamine and DMAP at –10°C for selective phosphate ester formation .
- Monoselective C–H alkylation : Catalyze ortho-alkylation of N-quinolyl benzamides with alkyl iodides, enabling precise functionalization of aromatic systems .
Q. What safety protocols are critical when handling this compound?
The compound is hazardous, causing skin/eye irritation and respiratory distress. Use fume hoods, nitrile gloves, and eye protection. Store at 2–8°C in airtight containers, avoiding oxidizers. Neutralize spills with sodium bicarbonate .
Advanced Research Questions
Q. How does this compound facilitate bioisosteric replacement in drug design?
As a phosphonate bioisostere, it replaces carboxylate or phosphate groups to enhance metabolic stability and bioavailability. For example, in prodrug synthesis (e.g., BNC105), this compound is used to create phosphate esters that improve solubility while maintaining target binding . Validate bioisosteric effects via molecular docking and in vitro assays comparing parent and modified compounds.
Q. What mechanistic insights explain its role in stereoselective synthesis?
In diastereoselective additions (e.g., Scheme 2 in ), this compound reacts with imines to form phosphonates. The bulky benzyl groups induce steric control, favoring specific diastereomers. Monitor reaction progress via ³¹P NMR to track intermediate formation .
Q. How can researchers resolve contradictions in reported synthetic yields?
Discrepancies in yields (e.g., 95% vs. lower values) often arise from catalyst purity, moisture levels, or temperature gradients. Replicate protocols rigorously using anhydrous solvents, fresh catalysts, and calibrated heating systems. Compare results with literature controls (e.g., PubChem data ).
Q. What advanced analytical methods ensure purity in this compound-based reactions?
Beyond NMR and MS, use high-performance liquid chromatography (HPLC) with UV detection at 254 nm to quantify residual reactants. For phosphonate products, employ ion chromatography to detect phosphate byproducts. Document purity criteria following Beilstein Journal guidelines (e.g., ≥95% purity for publication) .
Q. How is this compound applied in continuous flow chemistry?
In microwave-assisted alcoholysis (Table 5, ), continuous flow systems improve reaction efficiency by maintaining precise temperature control and reducing side reactions. Optimize parameters (e.g., flow rate, microwave power) using design-of-experiment (DoE) frameworks .
Q. What strategies mitigate challenges in prodrug activation using this compound?
For phosphate prodrugs (e.g., SCH-59884), this compound intermediates require hydrogenolysis to remove benzyl groups. Use Pd/C under H₂ or transfer hydrogenation with ammonium formate. Monitor deprotection via TLC and confirm with ¹H NMR (loss of benzyl protons at δ ~7.3 ppm) .
Q. Methodological Guidance
- Experimental Design : For reproducibility, document reaction parameters (e.g., stoichiometry, catalyst loading) and validate with triplicate runs. Use statistical tools (e.g., ANOVA) to assess variability .
- Data Reporting : Adhere to IUPAC nomenclature and include spectral data (NMR shifts, MS peaks) in supplementary materials. Reference PubChem identifiers (e.g., DTXSID80871270) for cross-validation .
Properties
Molecular Formula |
C14H14O3P- |
---|---|
Molecular Weight |
261.23 g/mol |
IUPAC Name |
dibenzyl phosphite |
InChI |
InChI=1S/C14H14O3P/c15-18(16-11-13-7-3-1-4-8-13)17-12-14-9-5-2-6-10-14/h1-10H,11-12H2/q-1 |
InChI Key |
GSNWGJLIRMGHAN-UHFFFAOYSA-N |
Canonical SMILES |
C1=CC=C(C=C1)COP([O-])OCC2=CC=CC=C2 |
Origin of Product |
United States |
Disclaimer and Information on In-Vitro Research Products
Please be aware that all articles and product information presented on BenchChem are intended solely for informational purposes. The products available for purchase on BenchChem are specifically designed for in-vitro studies, which are conducted outside of living organisms. In-vitro studies, derived from the Latin term "in glass," involve experiments performed in controlled laboratory settings using cells or tissues. It is important to note that these products are not categorized as medicines or drugs, and they have not received approval from the FDA for the prevention, treatment, or cure of any medical condition, ailment, or disease. We must emphasize that any form of bodily introduction of these products into humans or animals is strictly prohibited by law. It is essential to adhere to these guidelines to ensure compliance with legal and ethical standards in research and experimentation.