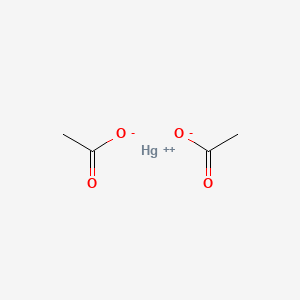
Mercury(II) acetate
- Click on QUICK INQUIRY to receive a quote from our team of experts.
- With the quality product at a COMPETITIVE price, you can focus more on your research.
Overview
Description
Mercury(II) acetate, also known as this compound, is a chemical compound with the formula Hg(O₂CCH₃)₂. It is the mercury(II) salt of acetic acid and appears as a white, water-soluble solid. This compound is commonly used as a reagent to generate organomercury compounds from unsaturated organic precursors .
Preparation Methods
Synthetic Routes and Reaction Conditions: Mercury(II) acetate can be synthesized by reacting mercuric oxide with acetic acid. The reaction is as follows: [ \text{HgO} + 2 \text{CH}_3\text{COOH} \rightarrow \text{Hg(CH}_3\text{COO)}_2 + \text{H}_2\text{O} ] This reaction involves the dissolution of mercuric oxide in glacial acetic acid, resulting in the formation of mercuric acetate and water .
Industrial Production Methods: In industrial settings, the production of mercuric acetate follows a similar synthetic route, ensuring the reaction conditions are optimized for large-scale production. The process involves careful control of temperature and concentration to maximize yield and purity .
Chemical Reactions Analysis
Types of Reactions: Mercury(II) acetate undergoes various types of chemical reactions, including:
Oxidation: It acts as an oxidizing agent in organic synthesis.
Substitution: It participates in substitution reactions, particularly in the formation of organomercury compounds.
Common Reagents and Conditions:
Oxymercuration-Demercuration: this compound is used in the oxymercuration of alkenes, where it adds across the carbon-carbon double bond to form an organomercury intermediate.
Reaction with Hydrogen Sulfide: In acetic acid solution, mercuric acetate reacts with hydrogen sulfide to precipitate mercuric sulfide.
Major Products:
Alcohols: Formed through oxymercuration-demercuration of alkenes.
Mercuric Sulfide: Formed through reaction with hydrogen sulfide.
Scientific Research Applications
Mercury(II) acetate has a wide range of applications in scientific research:
Chemistry: It is used as a reagent in organic synthesis, particularly in the formation of organomercury compounds.
Biology: It is employed in various biochemical assays and studies involving mercury compounds.
Industry: It is used in non-aqueous titration and as an oxidizing agent in various industrial processes.
Mechanism of Action
The mechanism of action of mercuric acetate involves its interaction with nucleophiles. In the oxymercuration of alkenes, mercuric acetate adds across the carbon-carbon double bond, forming an organomercury intermediate. This intermediate is then reduced to yield the final product, typically an alcohol . The molecular targets and pathways involved include the formation of cyclic mercurinium ions, which are key intermediates in the reaction .
Comparison with Similar Compounds
Mercuric Chloride (HgCl₂): Another mercury(II) compound used in organic synthesis and as a disinfectant.
Phenyl Mercuric Acetate (C₈H₈HgO₂): Used as a preservative and in the manufacture of pharmaceuticals.
Uniqueness of Mercuric Acetate: Mercury(II) acetate is unique due to its specific reactivity in oxymercuration-demercuration reactions, which allows for the selective formation of alcohols from alkenes without rearrangement. This selectivity and efficiency make it a valuable reagent in organic synthesis .
Properties
CAS No. |
592-63-2 |
---|---|
Molecular Formula |
Hg(CH3COO)2 Hg(C2H3O2)2 C4H6HgO4 |
Molecular Weight |
318.68 g/mol |
IUPAC Name |
mercury(2+);diacetate |
InChI |
InChI=1S/2C2H4O2.Hg/c2*1-2(3)4;/h2*1H3,(H,3,4);/q;;+2/p-2 |
InChI Key |
BRMYZIKAHFEUFJ-UHFFFAOYSA-L |
SMILES |
CC(=O)[O-].CC(=O)[O-].[Hg+2] |
Canonical SMILES |
CC(=O)[O-].CC(=O)[O-].[Hg+2] |
boiling_point |
Decomposes (EPA, 1998) |
density |
3.27 (EPA, 1998) |
melting_point |
352 to 356 °F (EPA, 1998) |
physical_description |
Mercury acetate is a white crystalline solid with an odor of vinegar. Sensitive to light. Density 3.25 g / cm3. Toxic by inhalation (dust, etc.) and by ingestion. Water soluble white crystals with vinegar odor; [CAMEO] |
vapor_pressure |
0.17 [mmHg] |
Origin of Product |
United States |
Retrosynthesis Analysis
AI-Powered Synthesis Planning: Our tool employs the Template_relevance Pistachio, Template_relevance Bkms_metabolic, Template_relevance Pistachio_ringbreaker, Template_relevance Reaxys, Template_relevance Reaxys_biocatalysis model, leveraging a vast database of chemical reactions to predict feasible synthetic routes.
One-Step Synthesis Focus: Specifically designed for one-step synthesis, it provides concise and direct routes for your target compounds, streamlining the synthesis process.
Accurate Predictions: Utilizing the extensive PISTACHIO, BKMS_METABOLIC, PISTACHIO_RINGBREAKER, REAXYS, REAXYS_BIOCATALYSIS database, our tool offers high-accuracy predictions, reflecting the latest in chemical research and data.
Strategy Settings
Precursor scoring | Relevance Heuristic |
---|---|
Min. plausibility | 0.01 |
Model | Template_relevance |
Template Set | Pistachio/Bkms_metabolic/Pistachio_ringbreaker/Reaxys/Reaxys_biocatalysis |
Top-N result to add to graph | 6 |
Feasible Synthetic Routes
Disclaimer and Information on In-Vitro Research Products
Please be aware that all articles and product information presented on BenchChem are intended solely for informational purposes. The products available for purchase on BenchChem are specifically designed for in-vitro studies, which are conducted outside of living organisms. In-vitro studies, derived from the Latin term "in glass," involve experiments performed in controlled laboratory settings using cells or tissues. It is important to note that these products are not categorized as medicines or drugs, and they have not received approval from the FDA for the prevention, treatment, or cure of any medical condition, ailment, or disease. We must emphasize that any form of bodily introduction of these products into humans or animals is strictly prohibited by law. It is essential to adhere to these guidelines to ensure compliance with legal and ethical standards in research and experimentation.