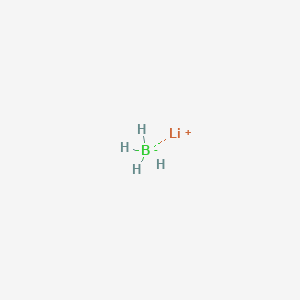
Lithium tetrahydroborate
- Click on QUICK INQUIRY to receive a quote from our team of experts.
- With the quality product at a COMPETITIVE price, you can focus more on your research.
Overview
Description
Lithium tetrahydroborate, also known as lithium tetrahydridoborate, is a chemical compound with the formula LiBH₄. It is a white crystalline solid that is highly soluble in ethers and reacts with water. This compound is known for its strong reducing properties and is commonly used in organic synthesis and as a hydrogen storage material.
Preparation Methods
Lithium tetrahydroborate can be synthesized through several methods. One common method involves the metathesis reaction between sodium borohydride and lithium bromide:
NaBH4+LiBr→NaBr+LiBH4
Another method involves the reaction of boron trifluoride with lithium hydride in diethyl ether:
BF3+4LiH→LiBH4+3LiF
These reactions are typically carried out under controlled conditions to ensure the purity and yield of the product.
Chemical Reactions Analysis
Lithium tetrahydroborate is a versatile reducing agent and can participate in various chemical reactions:
Reduction Reactions: It can reduce esters to alcohols, nitriles, and primary amides to amines, and can open epoxides.
Hydrogen Generation: It reacts with water to produce hydrogen gas, which can be used for hydrogen generation.
Reactions with Carbonyl Compounds: This compound can react with a range of carbonyl substrates and other polarized carbon structures to form hydrogen-carbon bonds.
Scientific Research Applications
Lithium tetrahydroborate has a wide range of applications in scientific research:
Biology: Its reducing properties are utilized in various biochemical applications, including the reduction of biomolecules.
Medicine: Research is ongoing into its potential use in drug synthesis and other pharmaceutical applications.
Mechanism of Action
The mechanism by which lithium boranuide exerts its effects is primarily through its ability to donate hydride ions (H⁻). This makes it a powerful reducing agent, capable of transferring electrons to other molecules and thereby reducing them. The lithium cation (Li⁺) also plays a role in stabilizing the intermediate complexes formed during these reactions .
Comparison with Similar Compounds
Lithium tetrahydroborate can be compared with other similar compounds such as:
Sodium borohydride (NaBH₄): While both are reducing agents, lithium boranuide is stronger and more reactive than sodium borohydride.
Lithium aluminium hydride (LiAlH₄): Lithium aluminium hydride is a more powerful reducing agent than lithium boranuide but is also more hazardous to handle.
Lithium tetrakis(pentafluorophenyl)borate: This compound is used in different applications, primarily in the preparation of cationic transition metal complexes.
This compound stands out due to its balance of reactivity and safety, making it a valuable reagent in both laboratory and industrial settings.
Properties
Molecular Formula |
BH4Li |
---|---|
Molecular Weight |
21.8 g/mol |
IUPAC Name |
lithium;boranuide |
InChI |
InChI=1S/BH4.Li/h1H4;/q-1;+1 |
InChI Key |
UUKMSDRCXNLYOO-UHFFFAOYSA-N |
Canonical SMILES |
[Li+].[BH4-] |
Origin of Product |
United States |
Disclaimer and Information on In-Vitro Research Products
Please be aware that all articles and product information presented on BenchChem are intended solely for informational purposes. The products available for purchase on BenchChem are specifically designed for in-vitro studies, which are conducted outside of living organisms. In-vitro studies, derived from the Latin term "in glass," involve experiments performed in controlled laboratory settings using cells or tissues. It is important to note that these products are not categorized as medicines or drugs, and they have not received approval from the FDA for the prevention, treatment, or cure of any medical condition, ailment, or disease. We must emphasize that any form of bodily introduction of these products into humans or animals is strictly prohibited by law. It is essential to adhere to these guidelines to ensure compliance with legal and ethical standards in research and experimentation.