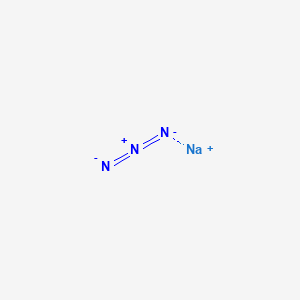
Sodium azide (Na(N3))
Overview
Description
Sodium azide is an inorganic compound with the chemical formula NaN3. It appears as a colorless to white crystalline solid and is highly soluble in water. Sodium azide is widely recognized for its use in automobile airbags, where it rapidly decomposes to produce nitrogen gas, inflating the airbag during a collision . Additionally, it is used in the synthesis of other azide compounds and serves as a preservative in laboratory reagents .
Preparation Methods
Sodium azide is commonly synthesized through the Wislicenus process, which involves two main steps in liquid ammonia . In the first step, metallic sodium reacts with ammonia to form sodium amide: [ 2 \text{Na} + 2 \text{NH}_3 \rightarrow 2 \text{NaNH}_2 + \text{H}_2 ] In the second step, sodium amide reacts with nitrous oxide to produce sodium azide: [ 2 \text{NaNH}_2 + \text{N}_2\text{O} \rightarrow \text{NaN}_3 + \text{NaOH} + \text{NH}_3 ]
Chemical Reactions Analysis
Sodium azide undergoes various types of chemical reactions, including:
Decomposition: When heated, sodium azide decomposes to form sodium and nitrogen gas[ 2 \text{NaN}_3 \rightarrow 2 \text{Na} + 3 \text{N}_2 ]
Reaction with Acids: Sodium azide reacts with strong acids to produce hydrazoic acid (HN3), which is highly toxic[ \text{H}^+ + \text{N}_3^- \rightarrow \text{HN}_3 ]
Nucleophilic Substitution: The azide ion (N3^-) is a strong nucleophile and can participate in nucleophilic substitution reactions with alkyl halides to form alkyl azides.
Scientific Research Applications
Mechanism of Action
Sodium azide exerts its effects primarily by inhibiting cytochrome oxidase, an enzyme involved in the electron transport chain of cellular respiration. By binding to the iron in the heme cofactor of cytochrome oxidase, sodium azide disrupts the electron transport chain, leading to a decrease in ATP production and an increase in reactive oxygen species (ROS) production . This can result in cellular apoptosis, particularly in neurons .
Comparison with Similar Compounds
Sodium azide is similar to other azide compounds, such as potassium azide (KN3) and ammonium azide (NH4N3). sodium azide is unique in its widespread use in airbags and its role as a preservative in laboratory reagents . Other similar compounds include:
Potassium Azide (KN3): Used in similar applications but less common than sodium azide.
Ammonium Azide (NH4N3): Used in explosives and propellants.
Magnesium Azide (Mg(N3)2): Used in specialized applications in the chemical industry.
Sodium azide’s unique properties and applications make it a valuable compound in various fields of science and industry.
Properties
Molecular Formula |
N3Na |
---|---|
Molecular Weight |
65.010 g/mol |
IUPAC Name |
sodium;azide |
InChI |
InChI=1S/N3.Na/c1-3-2;/q-1;+1 |
InChI Key |
PXIPVTKHYLBLMZ-UHFFFAOYSA-N |
Canonical SMILES |
[N-]=[N+]=[N-].[Na+] |
Origin of Product |
United States |
Synthesis routes and methods I
Procedure details
Synthesis routes and methods II
Procedure details
Disclaimer and Information on In-Vitro Research Products
Please be aware that all articles and product information presented on BenchChem are intended solely for informational purposes. The products available for purchase on BenchChem are specifically designed for in-vitro studies, which are conducted outside of living organisms. In-vitro studies, derived from the Latin term "in glass," involve experiments performed in controlled laboratory settings using cells or tissues. It is important to note that these products are not categorized as medicines or drugs, and they have not received approval from the FDA for the prevention, treatment, or cure of any medical condition, ailment, or disease. We must emphasize that any form of bodily introduction of these products into humans or animals is strictly prohibited by law. It is essential to adhere to these guidelines to ensure compliance with legal and ethical standards in research and experimentation.