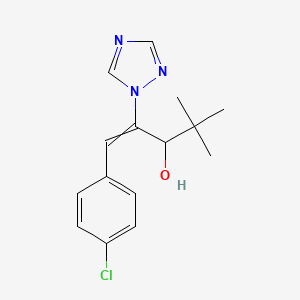
Uniconazole
Overview
Description
Uniconazole is a chemical compound with a complex structure that includes a triazole ring, a chlorophenyl group, and a tert-butyl group. This compound is known for its diverse applications in various fields, including chemistry, biology, medicine, and industry.
Preparation Methods
Synthetic Routes and Reaction Conditions
The synthesis of 1H-1,2,4-Triazole-1-ethanol, beta-[(4-chlorophenyl)methylene]-alpha-(1,1-dimethylethyl)- typically involves the reaction of 1H-1,2,4-triazole with an appropriate chlorophenyl derivative under controlled conditions. The reaction is often carried out in the presence of a base, such as sodium hydroxide, and a solvent, such as ethanol. The reaction mixture is heated to a specific temperature to facilitate the formation of the desired product .
Industrial Production Methods
In industrial settings, the production of this compound may involve more advanced techniques, such as continuous flow synthesis, to ensure high yield and purity. The use of automated reactors and precise control of reaction parameters can enhance the efficiency of the production process .
Chemical Reactions Analysis
Metabolism in Plants and Animals
Uniconazole undergoes distinct metabolic pathways in plants and animals, characterized by isomerization, conjugation, and enzymatic transformations.
Plant Metabolism
-
E/Z Isomerization : The major ES isomer undergoes reversible E/Z isomerization under physiological conditions .
-
Cyclization : Followed by hydroxylation at the terminal carbon and conjugation (e.g., formation of CYC-4Cl derivatives) .
-
Oxidation : In tomatoes, oxidation of the hydroxyl group to a ketone occurs .
Animal Metabolism
-
Rapid Absorption : Excreted primarily via feces (30–60%) and urine (40–67%) within 7 days .
-
Extensive Metabolism : ~80–90% of the dose is converted to biotransformation products, including CH₂OH-7E and COOH-7E .
-
Sex-Specific Differences : Males excrete slightly more via feces, with metabolites like triazole accumulating in blood .
Metabolic Pathways Comparison
Adsorption and Corrosion Inhibition on Steel Surfaces
This compound acts as a mixed-type corrosion inhibitor for steel in simulated concrete pore solutions.
Mechanism
-
Adsorption : Forms a hydrophobic layer on steel surfaces, reducing charge transfer and impeding corrosive interactions .
-
Electrochemical Behavior :
Surface Morphology
This compound Concentration | Surface Features |
---|---|
mol/L | Moderate reduction in corrosion pits |
mol/L | Smooth surface with minimal pitting |
Interaction with Chlorophyll a-b Binding Proteins
This compound binds to chlorophyll a-b binding protein 13 (Cab13), affecting photosynthetic efficiency under cold stress .
Binding Characteristics
-
Affinity : Confirmed via isothermal titration calorimetry (ITC) .
-
Impact : Alters photosynthetic pathways, particularly in mung bean under cold stress .
Modulation of Phenylpropanoid and Flavonoid Pathways
This compound represses lignin synthesis and alters flavonoid production by targeting key enzymes.
Key Pathways
-
Phenylpropanoid Biosynthesis : Inhibits BrbZIP39–BrPAL4 module, reducing lignin content .
-
Flavonoid Biosynthesis : Upregulates flavonoids (e.g., kaempferol-3,7-O-dirhamnoside) via phenylpropanoid pathway modulation .
Enzyme Activity Changes
Enzyme | Activity Trend (this compound-Treated vs. Control) |
---|---|
Phenylalanine Ammonia-Lyase (PAL) | ↓↓↓ (Hypocotyl dwarfing) |
Shikimate O-Hydroxycinnamoyltransferase (HCT) | ↑↑↑ (Banana dwarfism) |
Scientific Research Applications
Crop Yield Improvement
- Rice : Studies have demonstrated that exogenous application of Uniconazole significantly enhances the physiological metabolism and grain yield of rice under salt stress conditions. It improves stress tolerance by modulating morphogenesis and physiological processes, leading to better growth parameters and yield outcomes (Zhou et al., 2024) .
- Maize : this compound has been shown to increase maize yield by altering ear shape, enhancing photosynthetic efficiency, and boosting antioxidant activity under salt stress (Xu et al., 2022) .
- Rapeseed : Foliar application of this compound improves heat tolerance in rapeseed, allowing for better performance during high-temperature stress (Zhou and Leul, 1999) .
Stress Tolerance Enhancement
- Drought Resistance : In wheat, this compound application has been linked to improved drought tolerance by increasing leaf relative water content and reducing oxidative damage from reactive oxygen species (ROS). It enhances the activity of antioxidant enzymes and regulates gene expression related to stress responses (Zeng et al., 2023) .
- Salinity Stress : Studies indicate that this compound can mitigate the adverse effects of salinity on various crops by enhancing their physiological processes, leading to improved growth and productivity under saline conditions (Al-Rumaih, 2007) .
Physiological Effects
This compound affects several physiological parameters in plants:
- Photosynthetic Efficiency : The compound enhances chlorophyll content and promotes the expression of photosynthetic genes, thereby improving the overall photosynthetic capacity of plants under stress (Yu et al., 2022) .
- Antioxidant Activity : It increases the activity of antioxidant enzymes such as superoxide dismutase, which helps in scavenging ROS generated during stress conditions (Zeng et al., 2023) .
- Morphological Changes : The application leads to reduced plant height but increased root biomass, improving stability and nutrient uptake efficiency during adverse environmental conditions (Zhou et al., 2024) .
Case Studies
Mechanism of Action
The mechanism of action of 1H-1,2,4-Triazole-1-ethanol, beta-[(4-chlorophenyl)methylene]-alpha-(1,1-dimethylethyl)- involves its interaction with specific molecular targets and pathways. The triazole ring can bind to enzymes and receptors, modulating their activity. This interaction can lead to various biological effects, such as inhibition of microbial growth or modulation of cellular signaling pathways .
Comparison with Similar Compounds
Similar Compounds
1H-1,2,4-Triazole-1-ethanol, beta-[(2,4-dichlorophenyl)methyl]-alpha-(1,1-dimethylethyl)-: This compound has a similar structure but with two chlorine atoms on the phenyl ring.
Bitertanol: Another triazole derivative with a biphenyl group instead of a chlorophenyl group.
Uniconazole: A related compound with a similar triazole structure but different substituents.
Uniqueness
1H-1,2,4-Triazole-1-ethanol, beta-[(4-chlorophenyl)methylene]-alpha-(1,1-dimethylethyl)- is unique due to its specific combination of functional groups, which confer distinct chemical and biological properties.
Q & A
Basic Research Questions
Q. How can researchers quantify uniconazole concentrations in plant tissues and soil for experimental validation?
- Methodological Answer : Use high-performance liquid chromatography (HPLC) with methanol as the mobile phase for precise quantification. For example, a validated protocol achieved a recovery rate of 100.14% with a correlation coefficient of 0.9999, ensuring high accuracy . For soil and plant residue analysis, HPLC-tandem mass spectrometry (HPLC-MS/MS) is recommended, with dissipation half-lives of 4.2–5.0 days in cotton leaves and 15.8–19.7 days in soil . Include calibration curves and triplicate measurements to ensure reproducibility.
Q. What experimental design considerations are critical for studying this compound’s growth-retardant effects?
- Methodological Answer : Use a split-plot design with controlled variables (e.g., cutting types, concentrations). For example, terminal vs. subterminal cuttings of Jacobinia carnea showed divergent responses to this compound (60–180 ppm), with terminal cuttings exhibiting superior root development but delayed flowering . Apply frameworks like PICOT (Population, Intervention, Comparison, Outcome, Time) or FINER (Feasible, Interesting, Novel, Ethical, Relevant) to structure hypotheses and ensure ethical compliance .
Q. What are the optimal this compound concentrations for modulating plant stress responses without inducing toxicity?
- Methodological Answer : Conduct dose-response experiments with incremental concentrations (e.g., 20–800 mg/L). For drought resistance in wheat, 20–60 mg/L increased root-shoot ratios by 80–106% and chlorophyll content by 11–18.7%, while higher doses (>150 ppm) in Jacobinia carnea reduced root length and delayed flowering . Use ANOVA with Duncan’s post hoc tests (p < 0.05) to identify non-linear effects .
Q. How does this compound influence photosynthetic efficiency and chlorophyll biosynthesis?
- Methodological Answer : Measure chlorophyll a/b ratios and net photosynthetic rates under controlled light conditions. In duckweed (Landoltia punctata), 800 mg/L this compound increased chlorophyll content by 40% and altered gibberellin (GA) and abscisic acid (ABA) levels, linked to upregulated biosynthetic genes (e.g., CAO, HEMA) . Pair biochemical assays with transcriptome sequencing to identify regulatory pathways.
Q. What parameters should be monitored to assess this compound’s efficacy in drought resistance studies?
- Methodological Answer : Track malondialdehyde (MDA) content (a lipid peroxidation marker), relative water content, and osmolyte accumulation (e.g., proline, soluble sugars). In drought-stressed coix seedlings, this compound reduced MDA by 30% and enhanced superoxide dismutase (SOD) activity, indicating improved oxidative stress tolerance . Use triplicate biological replicates and standardized growth chambers to minimize environmental variability.
Advanced Research Questions
Q. How can researchers resolve contradictory findings on this compound’s carrier volume effects across plant species?
- Methodological Answer : Replicate experiments across species (e.g., chrysanthemums vs. poinsettias) under identical environmental conditions. For instance, carrier volume had no significant effect on efficacy in chrysanthemums, but species-specific cuticle permeability may explain discrepancies . Conduct meta-analyses of published data to identify confounding variables (e.g., humidity, surfactant use).
Q. What molecular mechanisms underlie this compound’s interaction with other plant growth regulators (PGRs)?
- Methodological Answer : Use transcriptome profiling and hormone quantification. In maize under cadmium stress, this compound (800 mg/L) synergized with GA₃ to increase root biomass by 69–123%, likely via competitive inhibition of GA biosynthesis . Pair RNA-seq data with enzyme activity assays (e.g., ent-kaurene oxidase) to validate pathway crosstalk.
Q. How does this compound affect long-term soil microbial communities and nutrient cycling?
- Methodological Answer : Monitor soil microbial diversity via 16S rRNA sequencing and enzyme activities (e.g., dehydrogenase, urease). In mango orchards, this compound reduced beneficial Actinobacteria abundance, necessitating multi-season field trials to assess recovery . Include untreated control plots and analyze residual this compound levels using HPLC-MS/MS .
Q. What experimental approaches can elucidate this compound’s enantiomer-specific metabolism in non-target organisms?
- Methodological Answer : Use chiral LC-MS/MS to track enantioselective accumulation. In rat liver microsomes, this compound enantiomers exhibited differential metabolic rates, highlighting the need for enantiomer-specific toxicity studies . Apply kinetic models (e.g., Michaelis-Menten) to compare metabolic pathways.
Q. How can transcriptome data be leveraged to optimize this compound application protocols for bioenergy crops?
- Methodological Answer : Integrate RNA-seq findings with physiological traits. In duckweed, this compound upregulated starch biosynthesis genes (AGPase, GBSS) by 2–5 fold, increasing starch yield by 50% . Validate candidate genes via CRISPR knockout lines and correlate expression levels with starch content under varying light regimes.
Q. Data Presentation and Statistical Standards
- Tables : Report means ± standard error (SE) with Duncan’s test annotations for significant differences (p < 0.05) .
- Figures : Use SigmaPlot or R for graphing, ensuring axis labels specify units (e.g., "Chlorophyll a (mg/g FW)") .
- Ethics : Disclose conflicts of interest and obtain permissions for reused data .
Properties
Molecular Formula |
C15H18ClN3O |
---|---|
Molecular Weight |
291.77 g/mol |
IUPAC Name |
1-(4-chlorophenyl)-4,4-dimethyl-2-(1,2,4-triazol-1-yl)pent-1-en-3-ol |
InChI |
InChI=1S/C15H18ClN3O/c1-15(2,3)14(20)13(19-10-17-9-18-19)8-11-4-6-12(16)7-5-11/h4-10,14,20H,1-3H3 |
InChI Key |
YNWVFADWVLCOPU-UHFFFAOYSA-N |
Canonical SMILES |
CC(C)(C)C(C(=CC1=CC=C(C=C1)Cl)N2C=NC=N2)O |
Origin of Product |
United States |
Synthesis routes and methods I
Procedure details
Synthesis routes and methods II
Procedure details
Synthesis routes and methods III
Procedure details
Disclaimer and Information on In-Vitro Research Products
Please be aware that all articles and product information presented on BenchChem are intended solely for informational purposes. The products available for purchase on BenchChem are specifically designed for in-vitro studies, which are conducted outside of living organisms. In-vitro studies, derived from the Latin term "in glass," involve experiments performed in controlled laboratory settings using cells or tissues. It is important to note that these products are not categorized as medicines or drugs, and they have not received approval from the FDA for the prevention, treatment, or cure of any medical condition, ailment, or disease. We must emphasize that any form of bodily introduction of these products into humans or animals is strictly prohibited by law. It is essential to adhere to these guidelines to ensure compliance with legal and ethical standards in research and experimentation.