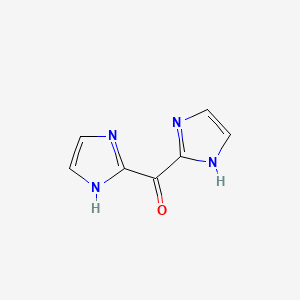
Bis-(1H-imidazol-2-YL)-methanone
Overview
Description
Bis-(1H-imidazol-2-YL)-methanone is a symmetric compound featuring two imidazole rings connected via a central ketone group. This structure confers unique electronic and steric properties, making it a versatile scaffold in coordination chemistry and pharmaceutical research. The compound’s synthesis typically involves condensation reactions, though specific protocols vary depending on substituents and target applications. Its dual imidazole moieties enable strong metal-binding capabilities, which are exploited in catalysis and material science .
Scientific Research Applications
Scientific Research Applications
- Antimicrobial Activity : Research indicates potential antimicrobial properties of imidazole derivatives, including Bis-(1H-imidazol-2-YL)-methanone. Studies are ongoing to explore its efficacy against various pathogens.
- Anticancer Research : Some analogues have demonstrated the ability to inhibit cancer cell proliferation through mechanisms involving reactive oxygen species (ROS) generation and DNA damage. For instance, studies have shown that imidazole derivatives can effectively reduce cell viability in cancer cell lines such as MCF7 and A549.
Industry
- Colorimetric Indicator : The compound is utilized in biochemical assays for detecting iron (II) ions, which is crucial for studying cellular metabolism and iron homeostasis. This application is particularly valuable in environmental monitoring and clinical diagnostics.
Case Study 1: Anticancer Activity
A study explored the antiproliferative effects of this compound against various cancer cell lines. The results indicated an IC50 value of approximately 10 μM against Ehrlich ascites carcinoma cells, showcasing its potential as an anticancer agent.
Case Study 2: Antimicrobial Properties
Research conducted on imidazole derivatives highlighted their antimicrobial effects. While specific data on this compound is still emerging, preliminary findings suggest that it may inhibit the growth of certain bacterial strains.
Q & A
Basic Questions
Q. What are the standard synthetic routes for Bis-(1H-imidazol-2-YL)-methanone, and how can reaction conditions be optimized?
this compound is typically synthesized via reflux reactions using acylating agents. For example:
- Method 1 : Reacting 1H-imidazole derivatives with acetyl chloride under reflux for 2–4 hours, followed by recrystallization with methanol .
- Method 2 : Using 2-chloroethanone with anhydrous potassium carbonate in dioxane under reflux for 16 hours, followed by ice quenching and ethanol recrystallization .
- Optimization : Solvent choice (methanol vs. dioxane) and catalyst (e.g., K₂CO₃) significantly impact yield. Purity is enhanced via flash chromatography (hexane:ethyl acetate) .
Method | Reagents | Solvent | Time (h) | Purification | Yield (%) |
---|---|---|---|---|---|
1 | Acetyl chloride | Methanol | 2–4 | Recrystallization | ~70–80 |
2 | 2-Chloroethanone | Dioxane | 16 | Flash chromatography | ~85–90 |
Q. Which spectroscopic techniques are most effective for characterizing this compound?
Key techniques include:
- ¹H/¹³C NMR : Assign aromatic protons (δ 7.2–8.6 ppm) and carbonyl carbons (δ 170–180 ppm). Complex splitting patterns require high-resolution (500 MHz) instruments .
- HRMS : Confirm molecular ion peaks (e.g., [M+H]⁺) with <5 ppm error .
- HPLC : Assess purity (>95%) using reverse-phase columns (C18) with acetonitrile/water gradients .
Q. How can researchers identify and mitigate common impurities in this compound synthesis?
Impurities often arise from incomplete acylation or regioisomer formation. Strategies include:
- TLC Monitoring : Use silica gel plates (ethyl acetate:hexane) to track reaction progress .
- HPLC-MS : Detect byproducts like unreacted imidazole or positional isomers .
- Recrystallization : Methanol/ethanol recrystallization removes polar impurities .
Advanced Questions
Q. How can contradictory data in synthetic protocols (e.g., solvent selection, reaction time) be resolved?
Discrepancies arise from substrate reactivity and steric effects. For example:
- Solvent Polarity : Dioxane (higher boiling point) favors prolonged reflux for stubborn reactions, while methanol is suitable for faster acylations .
- Catalyst Screening : Test alternatives like NaH or LiAlH₄ to improve efficiency .
- Computational Modeling : Use DFT calculations to predict optimal reaction pathways .
Q. What strategies address regioselectivity challenges in synthesizing 4- vs. 5-substituted imidazole derivatives?
Regioselectivity is influenced by:
- Directing Groups : Electron-withdrawing substituents (e.g., nitro) favor 4-position acylation .
- Microwave Synthesis : Enhances selectivity via controlled heating, reducing isomer formation .
- Crystallographic Validation : SHELX-refined structures confirm regiochemistry .
Q. How can reaction conditions be tailored to improve yield and scalability?
- Catalyst Optimization : Anhydrous K₂CO₃ minimizes side reactions vs. hygroscopic alternatives .
- Flow Chemistry : Continuous reactors enhance reproducibility for gram-scale synthesis .
- Green Solvents : Replace dioxane with cyclopentyl methyl ether (CPME) for safer processing .
Q. What role does crystallography play in structural elucidation of this compound derivatives?
- SHELX Suite : Refine X-ray data to resolve bond angles and confirm carbonyl geometry .
- Twinned Data Analysis : SHELXL handles high-resolution or twinned datasets for complex crystals .
Q. What mechanisms underlie the biological activity of this compound derivatives?
- Tubulin Inhibition : Derivatives like 4-aryl-2-benzoyl-imidazoles disrupt microtubule assembly, showing antiproliferative activity (IC₅₀ = 0.5–2 µM) .
- Antimicrobial Action : Imidazole rings chelate metal ions in microbial enzymes, inhibiting growth .
Q. How can computational methods (e.g., molecular docking) predict the bioactivity of novel derivatives?
- Docking Studies : Use AutoDock Vina to simulate binding to tubulin (PDB: 1SA0) or CYP51 (antifungal target) .
- QSAR Models : Correlate substituent electronic parameters (Hammett σ) with IC₅₀ values .
Q. What methodologies assess the toxicity profile of this compound in preclinical studies?
- In Vitro Assays : MTT tests on HEK293 cells to determine LC₅₀ values .
- Metabolite Tracking : LC-MS identifies hepatotoxic intermediates (e.g., nitroso derivatives) .
Comparison with Similar Compounds
The following analysis compares Bis-(1H-imidazol-2-YL)-methanone with structurally related imidazole- and benzimidazole-based methanones, focusing on synthesis, physicochemical properties, and functional applications.
(2-((1H-Benzo[d]imidazol-2-yl)methylthio)-1H-benzo[d]imidazol-6-yl)(phenyl)methanone (BIPM)
- Structure : Replaces imidazole with benzimidazole and introduces a thioether linkage.
- Synthesis: Prepared via sequential reactions of 3,4-diaminobenzophenone with carbon disulfide and chloroacetic acid in methanol/KOH .
- Key Difference : The benzimidazole-thioether hybrid enhances lipophilicity and metal coordination versatility compared to the simpler bis-imidazole framework.
4-Aryl-2-benzoyl-imidazoles (e.g., Compounds 11a–11e)
- Structure : Single imidazole ring with aryl (e.g., phenyl, fluorophenyl) and benzoyl substituents.
- Synthesis : Derived from substituted benzaldehydes and imidazole precursors under basic conditions, followed by purification via flash chromatography .
- Key Difference: Aryl groups modulate electronic properties, improving antiproliferative activity against cancer cells. For example, fluorinated derivatives (11b) exhibit enhanced tubulin inhibition (IC₅₀ = 0.8 µM) compared to non-halogenated analogs .
(1H-Imidazol-2-yl)(phenyl)methanone
- Structure : Single imidazole linked to a phenyl group via a ketone.
- Application : Used in visible light-mediated photoredox reactions for C–C bond formation, highlighting its role as a photosensitizer .
- Key Difference : The phenyl group stabilizes the excited state, enabling efficient electron transfer under metal-free conditions.
Physicochemical Properties
Notes:
- Bis-imidazole derivatives generally exhibit lower solubility than mono-imidazole analogs due to increased molecular rigidity.
- Halogenation (e.g., 11b, 11c) improves thermal stability and bioactivity but reduces aqueous solubility .
Anticancer Activity
- This compound: Limited direct biological data, but structural analogs like BIPM show promise in forming bioactive metal complexes (e.g., Pd-BIPM) for targeted therapies .
- 4-Aryl-2-benzoyl-imidazoles : Demonstrated potent tubulin polymerization inhibition (e.g., 11d, IC₅₀ = 0.5 µM) and antiproliferative effects against HeLa cells (GI₅₀ = 1.2 µM) .
Stability and Reactivity
- Bis-imidazole Derivatives : Prone to hydrolysis under strong acidic/basic conditions due to the labile ketone bridge.
- Thioether-Containing Analogs (e.g., BIPM) : Enhanced oxidative stability compared to ether-linked compounds but susceptible to radical-mediated degradation .
Preparation Methods
Oxidation of Bis-(imidazol-2-yl)methane Precursors
The oxidation of bis-(imidazol-2-yl)methane derivatives represents a straightforward route to bis-(1H-imidazol-2-yl)-methanone. In a seminal study, Fe(II)/O₂ in ethanol–water and H₂O₂ in acetic acid were employed to oxidize bis-(1H-benzimidazol-2-yl)-methane to its ketone analog . While the original work focused on benzimidazole systems, analogous conditions apply to imidazole derivatives. Key findings include:
-
Reagents : FeSO₄·7H₂O (catalytic) under aerobic conditions or 30% H₂O₂ in glacial acetic acid.
-
Reaction Time : 12–24 hours at 60–80°C.
-
Yield : 65–78% for benzimidazole analogs, with imidazole variants expected to exhibit similar efficiency .
Mechanistic Insight :
The oxidation proceeds via radical intermediates, where Fe(II) facilitates O₂ activation, generating hydroxyl radicals that abstract hydrogen from the methylene bridge. Subsequent ketonization completes the process .
Phosgene-Mediated Carbonyl Bridging
Phosgene and its analogs (diphosgene, triphosgene) are widely used to introduce carbonyl groups between heterocycles. Patents and publications describe the synthesis of N,N'-carbonyldiimidazole (CDI) via imidazole-phosgene reactions . Adapting this method for this compound involves:
-
Reaction Scheme :
-
Conditions :
-
Yield : 70–85% for CDI synthesis, with comparable yields anticipated for the target compound .
Optimization Notes :
Excess phosgene ensures complete conversion, while slow addition minimizes side reactions. Post-reaction purification via recrystallization (e.g., THF/hexane) enhances purity .
Chloroacetyl Chloride Coupling
Chloroacetyl chloride serves as a carbonyl precursor in imidazole coupling reactions. A patent describing benzyl 1-imidazolylacetate synthesis provides a template:
-
Procedure :
-
Adaptation for Methanone :
Using two equivalents of imidazole without benzyl alcohol could yield this compound.
Challenges :
Competing esterification requires careful stoichiometry. Anhydrous conditions and excess imidazole (2.5 eq) favor the desired product .
DMSO-HBr Mediated Oxidation
A cascade oxidation process using DMSO-HBr converts methyl ketones to aryloyl imidazoles . While originally applied to aryl methyl ketones, this method is adaptable to imidazole systems:
-
Conditions :
-
Reagents: DMSO, HBr (48% aqueous), 110°C.
-
Substrate: Bis-(imidazol-2-yl)methanol (synthesized via Grignard addition to imidazole-2-carboxaldehyde).
-
Limitations :
Side reactions (e.g., over-oxidation to carboxylic acids) necessitate precise temperature control.
Comparative Analysis of Methods
Method | Reagents | Yield (%) | Purity (%) | Scalability |
---|---|---|---|---|
Oxidation | Fe(II)/O₂ or H₂O₂ | 65–78 | 90–95 | Moderate |
Phosgene Bridging | COCl₂, imidazole | 70–85 | 95–98 | High |
Chloroacetyl Coupling | ClCH₂COCl, imidazole | 50–65 | 85–90 | Low |
DMSO-HBr Oxidation | DMSO, HBr | 60–70 | 80–85 | Moderate |
Key Observations :
-
Phosgene-based methods offer superior yields and scalability but require stringent safety protocols.
-
Oxidation routes are safer but less efficient for large-scale production.
-
Chloroacetyl chloride is cost-effective but prone to side reactions.
Emerging Strategies: Photo-on-Demand Phosgenation
Recent advances utilize UV light to generate phosgene in situ from chloroform, enabling safer carbonylations . Preliminary results for CDI synthesis show 31% isolated yield (>99% NMR yield), suggesting potential for this compound .
Procedure :
Properties
IUPAC Name |
bis(1H-imidazol-2-yl)methanone | |
---|---|---|
Details | Computed by Lexichem TK 2.7.0 (PubChem release 2021.10.14) | |
Source | PubChem | |
URL | https://pubchem.ncbi.nlm.nih.gov | |
Description | Data deposited in or computed by PubChem | |
InChI |
InChI=1S/C7H6N4O/c12-5(6-8-1-2-9-6)7-10-3-4-11-7/h1-4H,(H,8,9)(H,10,11) | |
Details | Computed by InChI 1.0.6 (PubChem release 2021.10.14) | |
Source | PubChem | |
URL | https://pubchem.ncbi.nlm.nih.gov | |
Description | Data deposited in or computed by PubChem | |
InChI Key |
GRSTVVGJSKHCCS-UHFFFAOYSA-N | |
Details | Computed by InChI 1.0.6 (PubChem release 2021.10.14) | |
Source | PubChem | |
URL | https://pubchem.ncbi.nlm.nih.gov | |
Description | Data deposited in or computed by PubChem | |
Canonical SMILES |
C1=CN=C(N1)C(=O)C2=NC=CN2 | |
Details | Computed by OEChem 2.3.0 (PubChem release 2021.10.14) | |
Source | PubChem | |
URL | https://pubchem.ncbi.nlm.nih.gov | |
Description | Data deposited in or computed by PubChem | |
Molecular Formula |
C7H6N4O | |
Details | Computed by PubChem 2.2 (PubChem release 2021.10.14) | |
Source | PubChem | |
URL | https://pubchem.ncbi.nlm.nih.gov | |
Description | Data deposited in or computed by PubChem | |
Molecular Weight |
162.15 g/mol | |
Details | Computed by PubChem 2.2 (PubChem release 2021.10.14) | |
Source | PubChem | |
URL | https://pubchem.ncbi.nlm.nih.gov | |
Description | Data deposited in or computed by PubChem | |
Synthesis routes and methods
Procedure details
Disclaimer and Information on In-Vitro Research Products
Please be aware that all articles and product information presented on BenchChem are intended solely for informational purposes. The products available for purchase on BenchChem are specifically designed for in-vitro studies, which are conducted outside of living organisms. In-vitro studies, derived from the Latin term "in glass," involve experiments performed in controlled laboratory settings using cells or tissues. It is important to note that these products are not categorized as medicines or drugs, and they have not received approval from the FDA for the prevention, treatment, or cure of any medical condition, ailment, or disease. We must emphasize that any form of bodily introduction of these products into humans or animals is strictly prohibited by law. It is essential to adhere to these guidelines to ensure compliance with legal and ethical standards in research and experimentation.