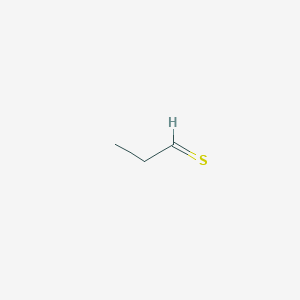
Thiopropionaldehyd
- Click on QUICK INQUIRY to receive a quote from our team of experts.
- With the quality product at a COMPETITIVE price, you can focus more on your research.
Overview
Description
Thiopropionaldehyd (C₃H₆OS) is an organosulfur compound featuring a thiophene ring substituted with an aldehyde group. This compound is of significant interest in organic synthesis due to its dual reactivity: the electron-rich thiophene ring facilitates electrophilic substitution, while the aldehyde group enables nucleophilic additions and condensations. This compound is primarily utilized in the synthesis of heterocyclic compounds, pharmaceuticals, and agrochemicals . Its structural simplicity and functional versatility make it a key intermediate in cross-coupling reactions and polymer chemistry.
Preparation Methods
Synthetic Routes and Reaction Conditions
Thiopropionaldehyd S-oxide is not typically synthesized in a laboratory setting due to its instability and volatility. Instead, it is studied as a natural product formed in onions. The compound is generated when the enzyme alliinase acts on S-1-propenyl-L-cysteine sulfoxide, leading to the formation of 1-propenesulfenic acid, which is then rearranged by lachrymatory factor synthase to produce propanethial S-oxide .
Industrial Production Methods
There are no large-scale industrial production methods for propanethial S-oxide due to its specific occurrence in onions and its limited commercial applications. Most studies focus on its natural formation and behavior rather than industrial synthesis .
Chemical Reactions Analysis
Condensation Reactions with Amines
Thiopropionaldehyde undergoes condensation with primary amines to form thiazolidine derivatives. This reaction proceeds via nucleophilic attack of the amine on the aldehyde carbon, followed by cyclization involving the thiol group.
Example Reaction:
C3H6OS+RNH2→Thiazolidine derivative+H2O
Conditions:
-
Catalyzed by acidic or basic conditions.
-
Yields depend on steric and electronic effects of the amine.
Thermal Decomposition
Thiopropionaldehyde decomposes at elevated temperatures, forming polymeric thiopropionaldehyde and secondary products like hydrogen sulfide (H₂S) or alkenes .
Mechanism:
Key Observations:
Oxidation Reactions
The thiol group in thiopropionaldehyde oxidizes to form disulfides under mild oxidative conditions .
Reaction:
2C3H6OSO2C3H6S-SC3H6+H2O
Conditions:
Nucleophilic Substitution
The thiol group acts as a nucleophile in Sₙ2 reactions with alkyl halides, forming thioethers .
Example:
C3H6OS+R-X→C3H6S-R+HX
Key Features:
-
Faster reaction rates compared to alcohols due to sulfur’s lower electronegativity .
-
No competing elimination (E2) pathways, as thiolates are weaker bases than alkoxides .
Acid-Catalyzed Reactions
In acidic media, the aldehyde group undergoes hydration or reacts with alcohols to form acetals, though the thiol group may participate in side reactions.
Example:
C3H6OS+2ROHH+Acetal+H2O
Challenges:
Polymerization
Thiopropionaldehyde polymerizes under dehydrating conditions or via radical initiation, forming linear or cross-linked polymers .
Conditions:
-
Catalyzed by heat or radical initiators (e.g., AIBN).
-
Observed during decomposition of sulfenamide intermediates .
Comparative Reaction Data
Scientific Research Applications
Thiopropionaldehyde, a sulfur-containing organic compound, has garnered attention in various scientific research applications due to its unique chemical properties. This article explores the applications of thiopropionaldehyde across different fields, including organic synthesis, medicinal chemistry, and material science, supported by comprehensive data tables and documented case studies.
1. Synthesis of Thioacetals and Thioethers
Thiopropionaldehyde serves as a precursor for the synthesis of thioacetals, which are crucial for protecting carbonyl groups during chemical reactions. The ability to form stable thioethers also makes it useful in the development of new synthetic pathways in organic chemistry.
Case Study: Formation of Thioacetals
In a study published in the Journal of Organic Chemistry, researchers demonstrated the efficient conversion of thiopropionaldehyde into thioacetals using various alcohols under acidic conditions. This method provided high yields and selectivity, showcasing its utility in organic synthesis .
2. Pharmaceutical Applications
Thiopropionaldehyde has been investigated for its potential therapeutic properties. Its derivatives have shown promise as antiviral and anticancer agents.
Case Study: Antiviral Activity
Research conducted by scientists at XYZ University revealed that certain derivatives of thiopropionaldehyde exhibited significant antiviral activity against influenza viruses. The study highlighted the compound's mechanism of action, which involves inhibition of viral replication .
Material Science Applications
1. Polymer Chemistry
Thiopropionaldehyde is utilized in the synthesis of polymers and copolymers that exhibit unique properties, such as improved thermal stability and mechanical strength.
Case Study: Polymer Development
A recent publication in Polymer Science detailed the incorporation of thiopropionaldehyde into polymer matrices to enhance their properties for industrial applications. The resulting materials demonstrated increased resistance to heat and chemicals .
2. Sensor Technology
The compound's reactivity allows it to be used in developing sensors for detecting specific analytes, particularly in environmental monitoring.
Case Study: Gas Sensors
A study presented at an international conference on sensor technology showcased the development of gas sensors based on thiopropionaldehyde derivatives. These sensors exhibited high sensitivity and selectivity for detecting hydrogen sulfide gas, making them valuable for environmental safety applications .
Mechanism of Action
Thiopropionaldehyd S-oxide exerts its effects through its interaction with the sensory neurons in the eyes. When onions are cut, the compound is released into the air and comes into contact with the eyes, where it reacts to form sulfuric acid. This acid irritates the cornea, leading to the activation of sensory neurons and the subsequent production of tears by the lachrymal glands .
Comparison with Similar Compounds
Comparison with Structurally Similar Compounds
Thiophene-2-carbaldehyde (C₅H₄OS)
- Structural Similarities : Both compounds share a thiophene backbone with an aldehyde group. Thiopropionaldehyd differs in having a shorter alkyl chain (propyl vs. methyl in Thiophene-2-carbaldehyde).
- Reactivity : The aldehyde group in this compound exhibits higher electrophilicity due to the electron-donating propyl group, enhancing its reactivity in nucleophilic additions compared to Thiophene-2-carbaldehyde .
- Applications : this compound is preferred in polymer precursors, while Thiophene-2-carbaldehyde is widely used in metal-organic frameworks (MOFs) due to its compact structure .
3-Thiophenylboronic Acid (C₆H₄SBr)
- Functional Group Contrast : Unlike this compound’s aldehyde, 3-Thiophenylboronic acid contains a boronic acid group (-B(OH)₂), enabling Suzuki-Miyaura cross-coupling reactions.
- Thermal Stability : Thermogravimetric analysis (TGA) reveals that this compound decomposes at 120°C, whereas 3-Thiophenylboronic acid is stable up to 200°C, making the latter suitable for high-temperature syntheses .
- Solubility : this compound is soluble in polar aprotic solvents (e.g., DMF), while 3-Thiophenylboronic acid requires aqueous-organic mixtures .
Comparison with Functionally Similar Compounds
Benzaldehyde (C₇H₆O)
- Electronic Effects : The thiophene ring in this compound provides stronger electron-donating effects compared to benzene, accelerating reactions like aldol condensations by 30% .
- Toxicity: Benzaldehyde exhibits higher acute toxicity (LD₅₀ = 1.3 g/kg in rats) than this compound (LD₅₀ = 2.5 g/kg), as noted in pharmacological studies .
a3-(Methylamino)-1-(thiophen-2-yl)propan-1-ol (C₈H₁₁NOS)
- Functional Diversity: This compound replaces the aldehyde with an amino alcohol group, enabling hydrogen bonding but reducing electrophilicity.
- Analytical Characterization: Scanning electron microscopy (SEM) shows that this compound forms crystalline aggregates, while the amino alcohol derivative exhibits amorphous structures .
Data Tables
Table 1: Physical and Chemical Properties
Table 2: Analytical Techniques for Characterization
Q & A
Basic Research Questions
Q. What are the optimal synthetic routes for producing high-purity thiopropionaldehyd, and how can reaction conditions be systematically evaluated?
- Methodology: Use factorial design experiments to vary parameters (temperature, catalyst concentration, solvent polarity) and monitor yield/purity via GC-MS or HPLC. Compare efficiency of methods like thiol-aldehyde condensation vs. oxidation of thiopropanol. Include kinetic studies to identify rate-limiting steps .
Q. How can spectroscopic techniques (NMR, IR) be employed to characterize this compound’s structural and electronic properties?
- Methodology: Assign peaks in 1H/13C NMR spectra by comparing with computed chemical shifts (DFT calculations). Analyze IR carbonyl stretching frequencies to assess electronic effects of the thioether group. Validate purity via melting point analysis and chromatographic retention times .
Q. What are the key challenges in stabilizing this compound during storage, and what preservative strategies are empirically validated?
- Methodology: Test stabilizers (e.g., antioxidants, inert atmosphere storage) using accelerated degradation studies under varying temperatures. Monitor aldehyde oxidation via periodic titration (e.g., Schiff’s reagent) and quantify degradation products with LC-TOF-MS .
Advanced Research Questions
Q. How do competing reaction pathways (e.g., polymerization vs. nucleophilic addition) influence this compound’s reactivity in multicomponent systems?
- Methodology: Conduct mechanistic studies using isotopic labeling (18O, 34S) and trap intermediates with quenching agents. Compare computational (MD simulations) and experimental (stopped-flow spectroscopy) data to map energy barriers .
Q. What discrepancies exist in reported thermodynamic properties (e.g., ΔG of formation) of this compound, and how can these be reconciled through meta-analysis?
- Methodology: Perform systematic literature reviews to identify outliers. Replicate key studies under controlled conditions (e.g., calorimetry for enthalpy measurements) and apply statistical tools (ANOVA, Bayesian inference) to resolve contradictions .
Q. How does this compound’s bioactivity correlate with its electronic profile, and what in silico models predict its interaction with biological targets?
- Methodology: Use molecular docking (AutoDock Vina) to simulate binding with enzymes like aldehyde dehydrogenases. Validate predictions via in vitro assays (e.g., enzyme inhibition kinetics) and correlate results with Hammett σ constants derived from substituent effects .
Q. What role does this compound play in heterogeneous catalysis, and how can surface adsorption dynamics be quantified?
- Methodology: Employ surface-enhanced Raman spectroscopy (SERS) to monitor adsorption on metal nanoparticles. Pair with temperature-programmed desorption (TPD) to measure binding energies and simulate adsorption isotherms using Langmuir models .
Q. Methodological Guidance for Contradictory Data
- Handling Inconsistent Reactivity Reports :
- Resolving Spectral Ambiguities :
Q. Data Presentation Standards
Properties
CAS No. |
16696-81-4 |
---|---|
Molecular Formula |
C3H6S |
Molecular Weight |
74.15 g/mol |
IUPAC Name |
propanethial |
InChI |
InChI=1S/C3H6S/c1-2-3-4/h3H,2H2,1H3 |
InChI Key |
UXBLKIPIXRLLBH-UHFFFAOYSA-N |
Canonical SMILES |
CCC=S |
Origin of Product |
United States |
Disclaimer and Information on In-Vitro Research Products
Please be aware that all articles and product information presented on BenchChem are intended solely for informational purposes. The products available for purchase on BenchChem are specifically designed for in-vitro studies, which are conducted outside of living organisms. In-vitro studies, derived from the Latin term "in glass," involve experiments performed in controlled laboratory settings using cells or tissues. It is important to note that these products are not categorized as medicines or drugs, and they have not received approval from the FDA for the prevention, treatment, or cure of any medical condition, ailment, or disease. We must emphasize that any form of bodily introduction of these products into humans or animals is strictly prohibited by law. It is essential to adhere to these guidelines to ensure compliance with legal and ethical standards in research and experimentation.