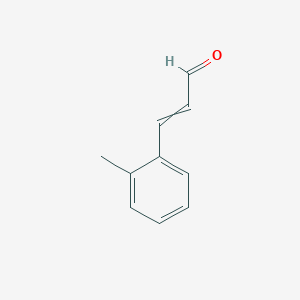
3-(o-Tolyl)acrylaldehyde
- Click on QUICK INQUIRY to receive a quote from our team of experts.
- With the quality product at a COMPETITIVE price, you can focus more on your research.
Overview
Description
3-(o-Tolyl)acrylaldehyde, also known as 3-(2-methylphenyl)propenal, is an organic compound characterized by the presence of a tolyl group attached to a propenal moiety. This compound is of significant interest in organic chemistry due to its unique structural features and reactivity.
Preparation Methods
Synthetic Routes and Reaction Conditions: The synthesis of 3-(o-Tolyl)acrylaldehyde typically involves the aldol condensation of o-tolualdehyde with acetaldehyde. The reaction is catalyzed by a base such as sodium hydroxide or potassium hydroxide. The reaction conditions include maintaining a temperature of around 0-5°C to control the reaction rate and prevent side reactions.
Industrial Production Methods: On an industrial scale, the production of this compound can be achieved through continuous flow reactors, which allow for better control of reaction parameters and higher yields. The use of catalysts such as zeolites or metal-organic frameworks (MOFs) can enhance the efficiency of the reaction.
Chemical Reactions Analysis
Types of Reactions: 3-(o-Tolyl)acrylaldehyde undergoes various types of chemical reactions, including:
Oxidation: The compound can be oxidized to form 3-o-Tolyl-propanoic acid using oxidizing agents such as potassium permanganate or chromium trioxide.
Reduction: Reduction of this compound can yield 3-o-Tolyl-propanol using reducing agents like sodium borohydride or lithium aluminum hydride.
Substitution: The aldehyde group in this compound can undergo nucleophilic substitution reactions with nucleophiles such as amines or alcohols to form imines or acetals, respectively.
Common Reagents and Conditions:
Oxidation: Potassium permanganate in an acidic medium.
Reduction: Sodium borohydride in methanol or ethanol.
Substitution: Primary amines or alcohols in the presence of an acid catalyst.
Major Products Formed:
Oxidation: 3-o-Tolyl-propanoic acid.
Reduction: 3-o-Tolyl-propanol.
Substitution: Imines or acetals depending on the nucleophile used.
Scientific Research Applications
3-(o-Tolyl)acrylaldehyde has a wide range of applications in scientific research:
Chemistry: It is used as an intermediate in the synthesis of various organic compounds, including pharmaceuticals and agrochemicals.
Biology: The compound is studied for its potential biological activities, including antimicrobial and anti-inflammatory properties.
Medicine: Research is ongoing to explore its potential as a precursor for drug development, particularly in the synthesis of anti-cancer agents.
Industry: this compound is used in the production of fragrances and flavoring agents due to its aromatic properties.
Mechanism of Action
The mechanism of action of 3-(o-Tolyl)acrylaldehyde involves its interaction with various molecular targets and pathways. The aldehyde group in the compound can form covalent bonds with nucleophilic sites in biological molecules, leading to the formation of Schiff bases. This interaction can modulate the activity of enzymes and receptors, thereby exerting its biological effects.
Comparison with Similar Compounds
3-p-Tolyl-propenal: Similar structure but with the tolyl group in the para position.
3-m-Tolyl-propenal: Similar structure but with the tolyl group in the meta position.
3-o-Tolyl-propanoic acid: The oxidized form of 3-(o-Tolyl)acrylaldehyde.
Uniqueness: this compound is unique due to the ortho position of the tolyl group, which influences its reactivity and the types of reactions it undergoes. This positional isomerism can lead to different chemical and biological properties compared to its meta and para counterparts.
Properties
CAS No. |
4549-82-0 |
---|---|
Molecular Formula |
C10H10O |
Molecular Weight |
146.19 g/mol |
IUPAC Name |
3-(2-methylphenyl)prop-2-enal |
InChI |
InChI=1S/C10H10O/c1-9-5-2-3-6-10(9)7-4-8-11/h2-8H,1H3 |
InChI Key |
ZAUAFFKABKNGKX-UHFFFAOYSA-N |
Canonical SMILES |
CC1=CC=CC=C1C=CC=O |
Origin of Product |
United States |
Disclaimer and Information on In-Vitro Research Products
Please be aware that all articles and product information presented on BenchChem are intended solely for informational purposes. The products available for purchase on BenchChem are specifically designed for in-vitro studies, which are conducted outside of living organisms. In-vitro studies, derived from the Latin term "in glass," involve experiments performed in controlled laboratory settings using cells or tissues. It is important to note that these products are not categorized as medicines or drugs, and they have not received approval from the FDA for the prevention, treatment, or cure of any medical condition, ailment, or disease. We must emphasize that any form of bodily introduction of these products into humans or animals is strictly prohibited by law. It is essential to adhere to these guidelines to ensure compliance with legal and ethical standards in research and experimentation.