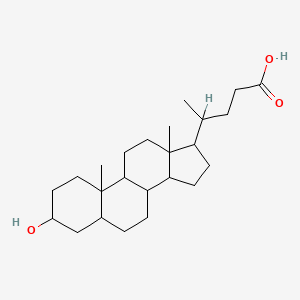
3-Hydroxycholan-24-oic acid
Overview
Description
3-Hydroxycholan-24-oic acid, commonly known as lithocholic acid (LCA), is a secondary bile acid with the molecular formula C₂₄H₄₀O₃ and a molecular weight of 376.57 g/mol . It is derived from the bacterial metabolism of chenodeoxycholic acid in the intestines and is notable for its hydrophobic nature due to a single hydroxyl group at the 3α-position of the steroid nucleus . LCA is primarily excreted in feces but is also conjugated in the liver to form taurolithocholic or glycolithocholic acids .
LCA has garnered attention for its role as a competitive antagonist of the EphA2 receptor, a tyrosine kinase implicated in cancer progression. It inhibits EphA2-ephrin-A1 interactions with a Ki of 49 ± 3 μM and blocks EphA2 autophosphorylation in prostate cancer cells . Despite its moderate potency, LCA serves as a scaffold for designing EphA2-targeting therapeutics .
Preparation Methods
Synthetic Routes and Reaction Conditions
The synthesis of 3-Hydroxycholan-24-oic acid typically involves the oxidation of cholic acid. The process includes several steps:
Oxidation: Cholic acid is oxidized using reagents such as chromium trioxide (CrO3) in acetic acid.
Reduction: The resulting product is then reduced using sodium borohydride (NaBH4) to yield deoxycholic acid.
Industrial Production Methods
Industrial production of deoxycholic acid involves the extraction from bovine bile. The bile is processed to isolate the acid, which is then purified through crystallization and other chemical processes .
Chemical Reactions Analysis
Types of Reactions
3-Hydroxycholan-24-oic acid undergoes various chemical reactions, including:
Oxidation: It can be oxidized to form keto derivatives.
Reduction: Reduction reactions can convert it back to its precursor forms.
Substitution: It can undergo substitution reactions at the hydroxyl groups.
Common Reagents and Conditions
Oxidizing Agents: Chromium trioxide (CrO3), potassium permanganate (KMnO4).
Reducing Agents: Sodium borohydride (NaBH4), lithium aluminum hydride (LiAlH4).
Solvents: Acetic acid, methanol.
Major Products
The major products formed from these reactions include various hydroxylated and keto derivatives, which have different biological and chemical properties .
Scientific Research Applications
Biochemical Functions
Lithocholic acid plays a crucial role in the digestion and absorption of dietary fats. As a bile acid, it acts as a detergent, facilitating the emulsification of lipids in the intestine. This property is vital for the absorption of fat-soluble vitamins and nutrients . Additionally, lithocholic acid is involved in the regulation of cholesterol metabolism and has been shown to influence the expression of genes related to lipid metabolism .
Cancer Research
Lithocholic acid has garnered attention for its potential anti-cancer properties. Preliminary studies suggest that it can selectively induce apoptosis in neuroblastoma cells while sparing normal neuronal cells. This selective cytotoxicity may be leveraged for therapeutic strategies against certain malignancies . Moreover, lithocholic acid's ability to modulate signaling pathways involved in cell proliferation and apoptosis makes it a candidate for further investigation in cancer therapeutics.
Metabolic Studies
Research indicates that lithocholic acid may play a role in metabolic disorders. Its interaction with the vitamin D receptor (VDR) has been studied, revealing that lithocholic acid can activate VDR without significantly increasing serum calcium levels, unlike vitamin D itself . This unique property positions lithocholic acid as a potential therapeutic agent for conditions like osteoporosis and metabolic syndrome.
Toxicological Studies
Lithocholic acid has been implicated in carcinogenesis, particularly in the colon. It can promote tumorigenesis through mechanisms such as inducing oxidative stress and altering gut microbiota composition . Understanding these toxicological effects is crucial for evaluating the safety of lithocholic acid in dietary supplements and pharmaceutical formulations.
Case Studies and Findings
Mechanism of Action
The compound exerts its effects primarily through its interaction with bile acids and lipid metabolism pathways. It acts by emulsifying fats, which aids in their absorption in the intestines. The molecular targets include various enzymes involved in lipid metabolism, such as lipases .
Comparison with Similar Compounds
Structural Comparison with Similar Bile Acids
Bile acids share a cholan-24-oic acid backbone but differ in hydroxylation and oxidation patterns, influencing their hydrophobicity and biological activity. Below is a comparative analysis of key bile acids (Table 1):
Table 1: Structural and Functional Comparison of Selected Bile Acids
EphA2 Receptor Antagonism
- LCA : Binds EphA2 via its hydrophobic core, disrupting ephrin-A1 interactions. The carboxylate group is critical for binding, while the 3α-OH group is dispensable .
- Cholanic Acid : Removal of the 3α-OH group enhances potency (Ki = 5.1 μM), highlighting the importance of hydrophobicity in EphA2 binding .
- Derivatives (e.g., UniPR126) : Conjugation of LCA with tryptophan improves antagonistic activity, demonstrating the role of structural modifications in enhancing efficacy .
Toxicity and Metabolism
- LCA is more hepatotoxic than other bile acids, causing cholestasis in high doses .
- Hydrophilic bile acids (e.g., α-MCA, HDCA) exhibit lower toxicity due to enhanced solubility and excretion .
Neuroprotective and Metabolic Roles
- LCA and its derivatives are implicated in neuroprotection , though mechanistic details remain understudied .
- Bile acids like DCA modulate BKCa channels , affecting cellular excitability and ion transport .
Structure-Activity Relationships (SAR)
- Hydroxyl Groups: 3α-OH (LCA): Non-essential for EphA2 binding but contributes to metabolic conjugation . Additional hydroxyls (e.g., DCA’s 12α-OH) reduce hydrophobicity and receptor affinity .
- Oxidation :
- Carboxylate Modifications :
- Esterification or conjugation (e.g., UniPR126) enhances potency by stabilizing salt bridges with EphA2’s Arg103 .
Q & A
Basic Research Questions
Q. What are the primary methods for isolating and characterizing 3-Hydroxycholan-24-oic acid from natural sources?
- Methodology : Isolation typically involves chromatographic techniques (e.g., column chromatography, HPLC) using plant or bile extracts, followed by structural elucidation via NMR (¹H and ¹³C), mass spectrometry (MS), and IR spectroscopy. For example, Allophylus longipes extracts yielded bile acid derivatives via silica gel chromatography and spectral analysis . Physicochemical properties, such as melting point (183–188°C) and specific rotation ([α]D²⁰ = +35.5° in ethanol), are critical for identification .
Q. What are the key physicochemical properties of LCA relevant to experimental design?
- Properties :
- Molecular formula: C₂₄H₄₀O₃; molecular weight: 376.57 .
- Solubility: Insoluble in water, soluble in methanol, ethanol, and dichloromethane .
- Stability: Store at 2–8°C in inert conditions to prevent degradation .
- Purity criteria: ≥98% by HPLC, ≤0.5% loss on drying, ≤20 ppm heavy metals .
Q. What is the biological significance of LCA in mammalian systems?
- Role : LCA is a secondary bile acid produced by gut microbiota, involved in lipid digestion and signaling. It modulates nuclear receptors (e.g., FXR, PXR) and has been implicated in liver function and cholesterol homeostasis . Elevated LCA levels are linked to cholestatic liver diseases, necessitating quantification via LC-MS in clinical research .
Advanced Research Questions
Q. How does LCA act as a competitive antagonist of the EphA2 receptor, and what are the implications for cancer research?
- Mechanism : LCA binds to the ligand-binding domain of EphA2, inhibiting ephrin-A1-induced receptor autophosphorylation (Ki = 49 ± 3 μM). This blocks downstream signaling pathways critical for tumor cell migration and angiogenesis . Functional assays in PC3 prostate cancer cells showed LCA inhibits EphA2-mediated cell retraction and rounding .
- Experimental Design : Use surface plasmon resonance (SPR) for binding kinetics (KD determination) and phospho-specific antibodies in Western blotting to assess EphA2 activation .
Q. How can structural modifications of LCA enhance its potency as an EphA2 antagonist?
- SAR Insights :
- The 3α-hydroxyl group is dispensable for receptor binding, while the carboxylate group at C-24 is critical for disrupting EphA2-ephrin-A1 interactions .
- Derivatives like (5β)-cholan-24-oic acid (cholanic acid) exhibit higher potency (lower μM range) due to optimized hydrophobic interactions .
Q. What experimental approaches resolve contradictions in LCA's reported bioactivity across studies?
- Case Example : Discrepancies in Ki values (e.g., 49 μM vs. lower values for derivatives) may arise from assay conditions (e.g., cell type, ligand concentration). Validate using orthogonal methods:
- Compare SPR-derived binding constants with cell-based functional assays (e.g., inhibition of EphA2 phosphorylation) .
- Control for batch-to-batch variability in LCA purity via HPLC and elemental analysis .
Q. How can researchers design in vitro assays to study LCA's interaction with serum albumin or other carriers?
- Methodology :
- Use fluorescence quenching assays to measure binding affinity to human serum albumin (HSA).
- Employ isothermal titration calorimetry (ITC) for thermodynamic profiling .
- Reference: Gadolinium-based contrast agent studies demonstrate albumin-binding assays for bile acid derivatives .
Q. Methodological Considerations
-
Synthesis & Purification :
-
Analytical Validation :
Properties
CAS No. |
516-73-4 |
---|---|
Molecular Formula |
C24H40O3 |
Molecular Weight |
376.6 g/mol |
IUPAC Name |
4-(3-hydroxy-10,13-dimethyl-2,3,4,5,6,7,8,9,11,12,14,15,16,17-tetradecahydro-1H-cyclopenta[a]phenanthren-17-yl)pentanoic acid |
InChI |
InChI=1S/C24H40O3/c1-15(4-9-22(26)27)19-7-8-20-18-6-5-16-14-17(25)10-12-23(16,2)21(18)11-13-24(19,20)3/h15-21,25H,4-14H2,1-3H3,(H,26,27) |
InChI Key |
SMEROWZSTRWXGI-UHFFFAOYSA-N |
SMILES |
CC(CCC(=O)O)C1CCC2C1(CCC3C2CCC4C3(CCC(C4)O)C)C |
Canonical SMILES |
CC(CCC(=O)O)C1CCC2C1(CCC3C2CCC4C3(CCC(C4)O)C)C |
Origin of Product |
United States |
Synthesis routes and methods
Procedure details
Retrosynthesis Analysis
AI-Powered Synthesis Planning: Our tool employs the Template_relevance Pistachio, Template_relevance Bkms_metabolic, Template_relevance Pistachio_ringbreaker, Template_relevance Reaxys, Template_relevance Reaxys_biocatalysis model, leveraging a vast database of chemical reactions to predict feasible synthetic routes.
One-Step Synthesis Focus: Specifically designed for one-step synthesis, it provides concise and direct routes for your target compounds, streamlining the synthesis process.
Accurate Predictions: Utilizing the extensive PISTACHIO, BKMS_METABOLIC, PISTACHIO_RINGBREAKER, REAXYS, REAXYS_BIOCATALYSIS database, our tool offers high-accuracy predictions, reflecting the latest in chemical research and data.
Strategy Settings
Precursor scoring | Relevance Heuristic |
---|---|
Min. plausibility | 0.01 |
Model | Template_relevance |
Template Set | Pistachio/Bkms_metabolic/Pistachio_ringbreaker/Reaxys/Reaxys_biocatalysis |
Top-N result to add to graph | 6 |
Feasible Synthetic Routes
Disclaimer and Information on In-Vitro Research Products
Please be aware that all articles and product information presented on BenchChem are intended solely for informational purposes. The products available for purchase on BenchChem are specifically designed for in-vitro studies, which are conducted outside of living organisms. In-vitro studies, derived from the Latin term "in glass," involve experiments performed in controlled laboratory settings using cells or tissues. It is important to note that these products are not categorized as medicines or drugs, and they have not received approval from the FDA for the prevention, treatment, or cure of any medical condition, ailment, or disease. We must emphasize that any form of bodily introduction of these products into humans or animals is strictly prohibited by law. It is essential to adhere to these guidelines to ensure compliance with legal and ethical standards in research and experimentation.