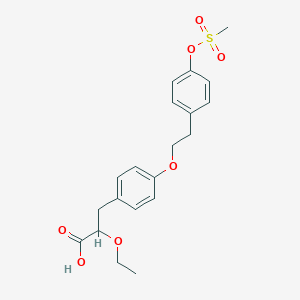
Tesaglitazar
Overview
Description
Tesaglitazar is a synthetic compound known for its dual-acting properties on peroxisome proliferator-activated receptors (PPARs). It is a white solid with a molecular formula of C20H24O7S and a molecular weight of 408.47 g/mol . This compound is primarily used in scientific research due to its unique chemical properties and biological activities.
Preparation Methods
Synthetic Routes and Reaction Conditions
The synthesis of Tesaglitazar involves multiple steps. One common method includes the use of 1,4-dioxane and benzenepropanamide as starting materials. The reaction proceeds through a series of steps involving the formation of intermediate compounds, which are then subjected to various reaction conditions to yield the final product .
Industrial Production Methods
Industrial production of this compound typically involves large-scale synthesis using optimized reaction conditions to ensure high yield and purity. The process may include the use of advanced techniques such as chromatography for purification and characterization of the final product .
Chemical Reactions Analysis
Types of Reactions
Tesaglitazar undergoes several types of chemical reactions, including:
Oxidation: This reaction involves the addition of oxygen or the removal of hydrogen, often using oxidizing agents like potassium permanganate.
Reduction: This reaction involves the addition of hydrogen or the removal of oxygen, typically using reducing agents like lithium aluminum hydride.
Substitution: This reaction involves the replacement of one functional group with another, often using reagents like halogens or alkylating agents.
Common Reagents and Conditions
Oxidation: Potassium permanganate in an acidic medium.
Reduction: Lithium aluminum hydride in anhydrous ether.
Substitution: Halogens or alkylating agents under controlled temperature and pressure conditions.
Major Products
The major products formed from these reactions depend on the specific conditions and reagents used. For example, oxidation may yield carboxylic acids, while reduction may produce alcohols .
Scientific Research Applications
Tesaglitazar has a wide range of applications in scientific research:
Chemistry: Used as a reagent in organic synthesis and as a standard in analytical chemistry.
Biology: Studied for its effects on cellular processes and signaling pathways.
Medicine: Investigated for its potential therapeutic effects, particularly in the treatment of metabolic disorders.
Industry: Utilized in the development of new materials and chemical products.
Mechanism of Action
The compound exerts its effects primarily through its action on PPARs, which are nuclear receptors involved in the regulation of gene expression. By binding to these receptors, the compound modulates the expression of genes involved in lipid metabolism, glucose homeostasis, and inflammation. This dual-acting property makes it a valuable tool in the study of metabolic diseases .
Comparison with Similar Compounds
Similar Compounds
Rosiglitazone: Another PPAR agonist used in the treatment of type 2 diabetes.
Pioglitazone: A PPAR agonist with similar therapeutic applications.
Fenofibrate: A PPAR agonist used to treat hyperlipidemia.
Uniqueness
Tesaglitazar is unique due to its dual-acting properties on both PPARα and PPARγ receptors. This dual action provides a broader range of effects compared to other PPAR agonists, making it a valuable compound for research and potential therapeutic applications .
Properties
CAS No. |
251565-88-5 |
---|---|
Molecular Formula |
C20H24O7S |
Molecular Weight |
408.5 g/mol |
IUPAC Name |
2-ethoxy-3-[4-[2-(4-methylsulfonyloxyphenyl)ethoxy]phenyl]propanoic acid |
InChI |
InChI=1S/C20H24O7S/c1-3-25-19(20(21)22)14-16-6-8-17(9-7-16)26-13-12-15-4-10-18(11-5-15)27-28(2,23)24/h4-11,19H,3,12-14H2,1-2H3,(H,21,22) |
InChI Key |
CXGTZJYQWSUFET-UHFFFAOYSA-N |
Canonical SMILES |
CCOC(CC1=CC=C(C=C1)OCCC2=CC=C(C=C2)OS(=O)(=O)C)C(=O)O |
Origin of Product |
United States |
Synthesis routes and methods I
Procedure details
Synthesis routes and methods II
Procedure details
Disclaimer and Information on In-Vitro Research Products
Please be aware that all articles and product information presented on BenchChem are intended solely for informational purposes. The products available for purchase on BenchChem are specifically designed for in-vitro studies, which are conducted outside of living organisms. In-vitro studies, derived from the Latin term "in glass," involve experiments performed in controlled laboratory settings using cells or tissues. It is important to note that these products are not categorized as medicines or drugs, and they have not received approval from the FDA for the prevention, treatment, or cure of any medical condition, ailment, or disease. We must emphasize that any form of bodily introduction of these products into humans or animals is strictly prohibited by law. It is essential to adhere to these guidelines to ensure compliance with legal and ethical standards in research and experimentation.