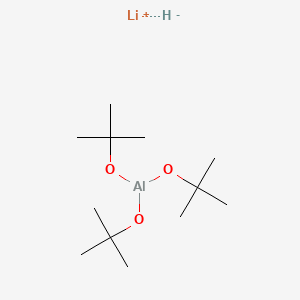
Lithium tri-tert-butoxyaluminum hydride
- Click on QUICK INQUIRY to receive a quote from our team of experts.
- With the quality product at a COMPETITIVE price, you can focus more on your research.
Overview
Description
Lithium tri-tert-butoxyaluminum hydride (LTBA, CAS: 17476-04-9) is a sterically hindered reducing agent derived from lithium aluminum hydride (LiAlH₄) by replacing three hydride groups with tert-butoxy ligands. Its molecular formula is C₁₂H₂₈AlLiO₃ (MW: 254.27 g/mol), and it exists as a white powder or colorless solution in tetrahydrofuran (THF) .
Preparation Methods
Classical Synthesis via Lithium Aluminum Hydride and Tert-Butanol
Reaction Overview
The classical method involves the controlled reaction of lithium aluminum hydride (LiAlH₄) with tert-butanol (t-BuOH) in an anhydrous tetrahydrofuran (THF) solvent. This approach capitalizes on the steric bulk of tert-butoxy groups to modulate the reactivity of the aluminum center, ensuring selective reductions in subsequent applications.
Stepwise Procedure
-
Dissolution of LiAlH₄ : Lithium aluminum hydride is dissolved in dry THF under an inert atmosphere (e.g., nitrogen or argon) to prevent moisture ingress.
-
Gradual Addition of Tert-Butanol : Tert-butanol is added dropwise at a temperature maintained between 0–5°C to mitigate exothermic side reactions.
-
Stirring and Reaction Completion : The mixture is stirred for 12–24 hours at room temperature to ensure complete substitution of hydride groups by tert-butoxy ligands.
-
Isolation and Purification : The product is filtered to remove unreacted LiAlH₄ or byproducts, followed by vacuum drying to yield LiAlH(Ot-Bu)₃ as a white crystalline solid.
Key Parameters
-
Molar Ratio : A 1:3 stoichiometric ratio of LiAlH₄ to t-BuOH is critical to avoid incomplete substitution.
-
Solvent Choice : THF’s low polarity and high stability under reducing conditions make it ideal for this reaction.
-
Yield : Typical yields range from 85% to 90%, with purity exceeding 95% after purification.
Alternative Cost-Effective Synthesis (Patent CN104725189A)
Three-Step Process Overview
This patented method reduces production costs by substituting metallic lithium with sodium and lithium chloride (LiCl) in the initial synthesis of lithium hydride (LiH) . The process comprises three stages:
Step 1: Synthesis of Lithium Hydride
-
Reagents : Sodium metal (99.5% purity), lithium chloride, and hydrogen gas .
-
Reaction Conditions :
2Na+LiCl+H2→LiH+2NaCl(ΔH<200∘C)The reaction occurs at 480–580°C, significantly lower than the 680–780°C required for traditional LiH synthesis from metallic lithium .
Step 2: Synthesis of Lithium Aluminum Hydride
-
Reagents : Lithium hydride (from Step 1) and aluminum chloride (AlCl₃) .
-
Reaction Conditions :
4LiH+AlCl3→LiAlH4+3LiCl(in diethyl ether, −10∘C)
Step 3: Synthesis of LiAlH(Ot-Bu)₃
-
Reagents : LiAlH₄ (from Step 2) and tert-butanol in methyl tert-butyl ether (MTBE) .
-
Reaction Conditions :
LiAlH4+3t-BuOH→LiAlH(Ot-Bu)3+3H2(0–10∘C, 24 hours)MTBE replaces traditional ethers, enhancing safety due to its higher flash point .
Advantages of the Patent Method
-
Cost Reduction : Sodium ($0.15/g) substitutes lithium ($3.00/g), lowering raw material costs by over 90% .
-
Safety Improvements : MTBE’s higher boiling point (55°C vs. 35°C for diethyl ether) reduces flammability risks .
-
Yield : Overall yield from LiCl to LiAlH(Ot-Bu)₃ exceeds 75%, comparable to classical methods .
Comparative Analysis of Preparation Methods
Chemical Reactions Analysis
Reduction of Acid Chlorides to Aldehydes
LiAlH(Ot-Bu)₃ is most widely used to reduce acid chlorides (RCOCl) to aldehydes (RCHO) under mild conditions. This reaction proceeds via nucleophilic acyl substitution , where the bulky tert-butoxy groups limit over-reduction to alcohols .
General Reaction:
RCOCl+LiAlH(Ot-Bu)3→RCHO+LiAl(Ot-Bu)3Cl
Mechanism:
-
Hydride Transfer : The hydride ion (H⁻) attacks the electrophilic carbonyl carbon of the acid chloride.
-
Elimination : The chloride ion (Cl⁻) is expelled, forming the aldehyde .
Key Features:
-
Superior to Rosenmund’s catalyst (Pd/BaSO₄ with H₂) due to fewer side reactions and compatibility with sensitive functional groups .
Reagent | Substrate | Product | Advantages |
---|---|---|---|
LiAlH(Ot-Bu)₃ | RCOCl | RCHO | Selective, avoids over-reduction; no H₂ gas needed |
Pd/BaSO₄ (Rosenmund) | RCOCl | RCHO | Requires H₂; risk of over-reduction |
Example:
Benzoyl chloride reduces to benzaldehyde in >90% yield at -78°C .
Reduction of Amides to Aldehydes
LiAlH(Ot-Bu)₃ enables the conversion of amides to aldehydes through imidate intermediates . This method is critical in synthesizing pharmaceuticals like gemcitabine .
Reaction Pathway:
-
Activation : Amides react with ethyl triflate (EtOTf) to form imidates.
-
Hydride Transfer : LiAlH(Ot-Bu)₃ reduces the imidate to a hemiaminal.
RCONR’2EtOTfRC(OR”)=NR’LiAlH(Ot-Bu)3RCH(OR”)NH2H2ORCHO
Conditions:
Scope:
Functional Group Selectivity
LiAlH(Ot-Bu)₃ exhibits remarkable chemoselectivity:
-
Reduces : Acid chlorides, amides, and ketones (under specific conditions) .
-
Inert Toward : Esters, nitriles, epoxides, and alkyl halides .
Comparison with LiAlH₄:
Reagent | Acid Chlorides | Esters | Amides | Nitriles |
---|---|---|---|---|
LiAlH(Ot-Bu)₃ | RCHO | No | RCHO | No |
LiAlH₄ | RCH₂OH | RCH₂OH | RCH₂NH₂ | RCH₂NH₂ |
Scientific Research Applications
Medicinal Chemistry
One of the most notable applications of lithium tri-tert-butoxyaluminum hydride is in the synthesis of pharmaceuticals. It has been utilized in the reduction of steroidal ketones, which are critical intermediates in the production of various therapeutic agents, including cancer treatments like gemcitabine .
Case Study: Synthesis of Gemcitabine
- Process : The reduction of a steroidal ketone using this compound was demonstrated to yield gemcitabine effectively.
- Outcome : This method provided a high-selectivity reduction pathway that minimized side reactions, which are common with more aggressive reducing agents like lithium aluminum hydride.
Organic Synthesis
This compound is extensively used in organic synthesis for the selective reduction of carbonyl compounds, including esters and amides.
Applications:
- Reduction of Acid Halides : It selectively reduces acid halides to aldehydes without affecting other functional groups . This selectivity is crucial in multi-step syntheses where maintaining functional group integrity is necessary.
- Hydroboration Reactions : The compound has been employed as a catalyst in hydroboration reactions, facilitating the conversion of alkenes to alkyl boronates efficiently .
Reaction Type | Reactants | Products | Selectivity |
---|---|---|---|
Reduction of Acid Halides | Acid Halide + LiAlH(O-t-Bu)3 | Aldehyde | High |
Hydroboration | Alkene + Pinacolborane | Alkyl Boronate | Good Yield |
Agricultural Chemicals
In the field of agricultural chemistry, this compound has been identified as a useful reagent for synthesizing agrochemicals. Its ability to selectively reduce ketones and esters makes it valuable for creating active ingredients in pesticides and herbicides .
Industrial Applications
The compound's utility extends into industrial applications where it serves as a reducing agent in various chemical processes. Its stability and selectivity make it a preferred choice over more reactive alternatives that may lead to unwanted by-products.
Mechanism of Action
The mechanism of action of lithium tri(tert-butoxy)aluminum hydride involves nucleophilic acyl substitution. The hydride ion from the compound attacks the carbonyl carbon of the substrate, leading to the formation of an intermediate, which then eliminates a leaving group to form the final reduced product . The bulky tert-butoxy groups help modulate the reactivity, ensuring selective reduction .
Comparison with Similar Compounds
Key Properties:
- Reactivity : LTBA is a mild and selective reductant , ideal for partial reductions (e.g., esters/amides to aldehydes) without over-reduction to alcohols .
- Thermal Stability : Operates effectively under reflux conditions (e.g., 66°C in THF) .
- Handling : Highly moisture-sensitive; requires inert atmosphere packaging (argon/vacuum) and storage at 2–8°C .
- Safety : Classified as hazardous (H225, H260, H314) due to flammability and corrosive reactivity with water .
Comparison with Similar Reducing Agents
Lithium Aluminum Hydride (LiAlH₄)
- Reactivity : Stronger and less selective than LTBA. Reduces esters, amides, and nitriles to alcohols, often causing over-reduction .
- Selectivity : Poor in multi-functional substrates. For example, LiAlH₄ reduces both ketones and phthalimido carbonyls, while LTBA selectively targets ketones .
- Applications: Broad utility in non-selective reductions.
- Safety : More pyrophoric and hazardous than LTBA .
Diisobutylaluminum Hydride (DIBAL-H)
- Reactivity : Similar steric bulk to LTBA but less oxophilic. Effective for partial reductions of esters to aldehydes .
- Selectivity : Prefers esters over amides; LTBA shows better compatibility with fluorinated systems .
- Handling : Typically used in toluene/hexane, whereas LTBA is THF-soluble .
Sodium Borohydride (NaBH₄)
- Reactivity : Milder than LTBA; ineffective for amides or fluorinated imidoyl chlorides .
- Selectivity: Limited to aldehydes/ketones; unsuitable for bulky substrates.
- Cost : Cheaper but less versatile in complex syntheses .
Lithium Triethylborohydride (Super Hydride)
- Reactivity : Stronger than LTBA but generates complex mixtures in stereoselective reductions .
- Applications : Useful for ketone reductions but less effective in diastereoselective protocols compared to LTBA .
Comparative Data Table
Parameter | LTBA | LiAlH₄ | DIBAL-H | NaBH₄ |
---|---|---|---|---|
Reactivity | Moderate | Very High | Moderate-High | Low |
Selectivity | High (aldehydes/ketones) | Low | Moderate | Low |
Typical Applications | Fluorinated systems, esters→aldehydes | Broad reductions | Esters→aldehydes | Aldehydes/ketones |
Handling | Moisture-sensitive (THF) | Pyrophoric | Toluene/hexane | Aqueous/organic |
Safety Hazards | Flammable, corrosive (H225, H314) | Extreme (F, C) | Flammable (F) | Low risk |
Research Findings and Case Studies
- Fluorinated Systems : LTBA efficiently reduces polyfluoroalkyl imidoyl chlorides to aldimines, outperforming NaBH₄, LiAlH₄, and DIBAL-H .
- Steroid Synthesis : LTBA enabled selective ketone reduction in steroidal intermediates without epimerization, critical for pharmaceutical applications .
- Diastereoselectivity Challenges : In one study, LTBA showed low conversion in titanium-mediated reductions, prompting use of NaBH₄ despite similar selectivity .
Q & A
Basic Research Questions
Q. How is LTBA prepared, and what factors influence its reactivity in reduction reactions?
LTBA is synthesized by reacting lithium aluminum hydride (LAH) with three equivalents of <i>tert</i>-butanol in anhydrous ether or tetrahydrofuran (THF) under nitrogen . Its reactivity is modulated by steric bulk from the <i>tert</i>-butoxy groups, which reduces its nucleophilicity compared to LAH. This makes LTBA selective for partial reductions (e.g., converting acid chlorides to aldehydes without over-reduction to alcohols). Key factors include solvent polarity (THF enhances solubility and reactivity) and temperature (room temperature suffices for most reactions) .
Q. What are the standard applications of LTBA in carbonyl reductions?
LTBA is commonly used to reduce acid chlorides to aldehydes, a reaction where LAH or NaBH₄ would over-reduce to primary alcohols . For example, isovaleroyl chloride is reduced to isovaleraldehyde in 65% yield using LTBA in THF . It also selectively reduces esters to alcohols while preserving other functional groups like nitriles or epoxides, as demonstrated in the synthesis of aldimines from fluorinated imidoyl chlorides .
Q. How does LTBA compare to DIBAL-H in terms of selectivity and reaction conditions?
LTBA and DIBAL-H both exhibit steric hindrance-driven selectivity, but LTBA is milder and less prone to side reactions with sensitive substrates. For instance, LTBA reduces polyfluoroalkyl imidoyl chlorides to aldimines efficiently, while DIBAL-H shows poor reactivity in the same systems . Reaction conditions for LTBA typically involve ambient temperatures and aprotic solvents like THF, whereas DIBAL-H may require lower temperatures for controlled reductions .
Advanced Research Questions
Q. What mechanistic insights explain LTBA’s unexpected stereoselectivity in cyclic ketone reductions?
Despite its steric bulk, LTBA exhibits lower stereoselectivity than smaller alkoxyaluminum hydrides (e.g., lithium trimethoxyaluminum hydride) in some systems. Studies suggest that LTBA may dissociate into a smaller active species, such as di-<i>tert</i>-butoxyaluminum hydride, during reactions, altering the transition state geometry. This hypothesis is supported by kinetic data showing non-linear relationships between LTBA concentration and reaction rates .
Q. How can researchers optimize LTBA-mediated reductions to address conflicting stereochemical outcomes?
Conflicting stereoselectivity data (e.g., axial vs. equatorial alcohol formation in epoxide reductions) can arise from solvent effects or substrate-LTBA coordination modes. To optimize:
- Use polar solvents (THF) to stabilize ion-pair intermediates.
- Pre-coordinate LTBA with Lewis acids (e.g., CeCl₃) to enhance selectivity.
- Monitor reaction progress via <sup>27</sup>Al NMR to identify active species .
Q. What strategies resolve contradictions in LTBA’s reactivity toward α,β-unsaturated carbonyl compounds?
LTBA may reduce α,β-unsaturated esters to allylic alcohols or leave the double bond intact, depending on substrate electronics. For example:
- Electron-deficient enones undergo 1,2-reduction.
- Electron-rich systems favor 1,4-reduction. Experimental validation via deuterium-labeling studies or computational modeling (DFT) can clarify the dominant pathway .
Q. How is LTBA employed in multi-step syntheses of complex molecules?
In the synthesis of racemic 13-ethyl-18,19-dinor-17a-pregn-4-en-20-yne-3β,17-diol, LTBA reduced a ketone intermediate in THF at room temperature over 18 hours, followed by chromatographic purification (5% ethyl acetate in benzene) to isolate the product in high yield . Critical steps include strict anhydrous conditions and quenching with aqueous NH₄Cl to prevent side reactions .
Q. Methodological Considerations
Q. What safety protocols are essential for handling LTBA in the laboratory?
LTBA is moisture-sensitive and reacts violently with water. Standard protocols include:
- Use of inert atmosphere (N₂/Ar) gloveboxes.
- Quenching with dry ice or isopropanol before aqueous workup.
- Storage in sealed, desiccated containers at –20°C .
Q. How can researchers validate the purity and activity of LTBA batches?
- Titration : React LTBA with a known excess of benzaldehyde and measure unreacted aldehyde via GC-MS.
- FT-IR : Confirm the presence of Al-H bonds (≈1800 cm⁻¹).
- Elemental analysis : Verify Li/Al ratios (theoretical: 1:1) .
Q. Data Contradiction Analysis
Q. Why do some studies report LTBA as less selective than its smaller analogs?
Contradictions arise from differences in substrate steric environments and solvent coordination. For example, LTBA’s bulk may hinder access to planar carbonyl groups but favor axial attack in cyclic ketones. Mechanistic studies using kinetic isotope effects (KIEs) and computational models (e.g., DFT) are recommended to resolve such discrepancies .
Properties
Molecular Formula |
C12H28AlLiO3 |
---|---|
Molecular Weight |
254.3 g/mol |
IUPAC Name |
lithium;hydride;tris[(2-methylpropan-2-yl)oxy]alumane |
InChI |
InChI=1S/3C4H9O.Al.Li.H/c3*1-4(2,3)5;;;/h3*1-3H3;;;/q3*-1;+3;+1;-1 |
InChI Key |
HCDSTARONIHDMB-UHFFFAOYSA-N |
Canonical SMILES |
[H-].[Li+].CC(C)(C)O[Al](OC(C)(C)C)OC(C)(C)C |
Origin of Product |
United States |
Disclaimer and Information on In-Vitro Research Products
Please be aware that all articles and product information presented on BenchChem are intended solely for informational purposes. The products available for purchase on BenchChem are specifically designed for in-vitro studies, which are conducted outside of living organisms. In-vitro studies, derived from the Latin term "in glass," involve experiments performed in controlled laboratory settings using cells or tissues. It is important to note that these products are not categorized as medicines or drugs, and they have not received approval from the FDA for the prevention, treatment, or cure of any medical condition, ailment, or disease. We must emphasize that any form of bodily introduction of these products into humans or animals is strictly prohibited by law. It is essential to adhere to these guidelines to ensure compliance with legal and ethical standards in research and experimentation.