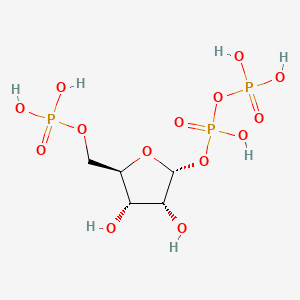
Alpha-Phosphoribosylpyrophosphoric Acid
- Click on QUICK INQUIRY to receive a quote from our team of experts.
- With the quality product at a COMPETITIVE price, you can focus more on your research.
Overview
Description
Preparation Methods
Synthetic Routes and Reaction Conditions
Alpha-Phosphoribosylpyrophosphoric Acid is synthesized from ribose 5-phosphate through the action of the enzyme ribose-phosphate diphosphokinase. This enzyme catalyzes the transfer of a pyrophosphate group from adenosine triphosphate (ATP) to ribose 5-phosphate, forming this compound .
Industrial Production Methods
In industrial settings, the production of this compound typically involves microbial fermentation processes. Genetically engineered microorganisms, such as Escherichia coli, are often used to overproduce the enzyme ribose-phosphate diphosphokinase, thereby increasing the yield of this compound .
Chemical Reactions Analysis
Types of Reactions
Alpha-Phosphoribosylpyrophosphoric Acid undergoes various biochemical reactions, including:
Phosphorylation: It can be phosphorylated to form different nucleotide derivatives.
Substitution: It participates in substitution reactions where the pyrophosphate group is replaced by other functional groups.
Common Reagents and Conditions
Conditions: These reactions typically occur under physiological conditions, with optimal temperatures around 37°C and neutral pH levels.
Major Products
The major products formed from reactions involving this compound include various nucleotides such as adenosine monophosphate (AMP), guanosine monophosphate (GMP), and inosine monophosphate (IMP) .
Scientific Research Applications
Alpha-Phosphoribosylpyrophosphoric Acid has a wide range of applications in scientific research:
Chemistry: It is used as a precursor in the synthesis of nucleotide analogs and other biochemical compounds.
Biology: It plays a vital role in the study of metabolic pathways involving nucleotide biosynthesis.
Mechanism of Action
Alpha-Phosphoribosylpyrophosphoric Acid exerts its effects by acting as a substrate for various enzymes involved in nucleotide biosynthesis. It transfers phospho-ribose groups to different acceptor molecules, facilitating the formation of nucleotides. The molecular targets include enzymes such as adenine phosphoribosyltransferase and hypoxanthine-guanine phosphoribosyltransferase, which are involved in the salvage pathways of nucleotide synthesis .
Comparison with Similar Compounds
Similar Compounds
Ribose 5-phosphate: A precursor in the synthesis of Alpha-Phosphoribosylpyrophosphoric Acid.
Adenosine triphosphate (ATP): Provides the pyrophosphate group in the synthesis of this compound.
Inosine monophosphate (IMP): A nucleotide formed from this compound.
Uniqueness
This compound is unique due to its central role in the biosynthesis of both purine and pyrimidine nucleotides. Unlike other similar compounds, it serves as a common precursor for a wide range of nucleotide derivatives, making it indispensable in nucleotide metabolism .
If you have any more questions or need further details, feel free to ask!
Biological Activity
Alpha-Phosphoribosylpyrophosphoric acid (PRPP) is a crucial metabolite involved in various biological processes, particularly in nucleotide biosynthesis. This article reviews the biological activity of PRPP, highlighting its role in metabolic pathways, enzymatic functions, and potential implications for health and disease.
Overview of this compound
PRPP is a pentose phosphate derivative that serves as a substrate for several key enzymes in nucleotide metabolism. It is synthesized from ribose-5-phosphate and ATP by the enzyme ribose-phosphate pyrophosphokinase (PRPP synthetase). The structure of PRPP features a ribose sugar linked to two phosphate groups, making it highly reactive.
Biological Functions
- Nucleotide Biosynthesis : PRPP is essential for the synthesis of purine and pyrimidine nucleotides. It acts as a ribosyl donor in the salvage pathways for nucleotides, facilitating the conversion of bases like adenine and guanine into their corresponding nucleotides (AMP and GMP) through phosphoribosyltransferase enzymes .
- Amino Acid Biosynthesis : PRPP also plays a role in the biosynthesis of certain amino acids, including histidine and tryptophan. The enzyme ATP phosphoribosyltransferase catalyzes the first step in histidine biosynthesis using PRPP as a substrate .
- Regulatory Role : The levels of PRPP can influence the activity of various enzymes involved in nucleotide synthesis, thus playing a regulatory role in cellular metabolism. For instance, high concentrations of PRPP can stimulate the activity of amidophosphoribosyltransferase, an enzyme critical for purine biosynthesis .
Case Study 1: PRPP and Cancer Metabolism
Recent studies have investigated the role of PRPP in cancer cell metabolism. Elevated levels of PRPP have been observed in certain cancer types, suggesting that tumor cells may exploit PRPP to enhance nucleotide synthesis for rapid proliferation. Inhibition of PRPP synthetase has been proposed as a therapeutic strategy to limit tumor growth by disrupting nucleotide supply .
Case Study 2: Genetic Disorders
Mutations affecting enzymes involved in PRPP metabolism can lead to various genetic disorders. For example, adenosine deaminase deficiency results in an accumulation of toxic metabolites due to impaired purine metabolism, highlighting the importance of PRPP in maintaining nucleotide balance within cells .
Data Table: Enzymatic Reactions Involving PRPP
Enzyme | Reaction Type | Products | Notes |
---|---|---|---|
Hypoxanthine-guanine phosphoribosyltransferase | Transfer | IMP, GMP | Central to purine salvage pathway |
Adenine phosphoribosyltransferase | Transfer | AMP | Important for recycling adenine |
Anthranilate phosphoribosyltransferase | Transfer | PRA | Involved in tryptophan biosynthesis |
ATP phosphoribosyltransferase | Condensation | N'-5'-phosphoribosyl-ATP | First step in histidine biosynthesis |
Properties
CAS No. |
13270-65-0 |
---|---|
Molecular Formula |
C5H13O14P3 |
Molecular Weight |
390.07 g/mol |
IUPAC Name |
[(2R,3R,4S,5R)-3,4-dihydroxy-5-(phosphonooxymethyl)oxolan-2-yl] phosphono hydrogen phosphate |
InChI |
InChI=1S/C5H13O14P3/c6-3-2(1-16-20(8,9)10)17-5(4(3)7)18-22(14,15)19-21(11,12)13/h2-7H,1H2,(H,14,15)(H2,8,9,10)(H2,11,12,13)/t2-,3-,4-,5-/m1/s1 |
InChI Key |
PQGCEDQWHSBAJP-TXICZTDVSA-N |
SMILES |
C(C1C(C(C(O1)OP(=O)(O)OP(=O)(O)O)O)O)OP(=O)(O)O |
Isomeric SMILES |
C([C@@H]1[C@H]([C@H]([C@H](O1)OP(=O)(O)OP(=O)(O)O)O)O)OP(=O)(O)O |
Canonical SMILES |
C(C1C(C(C(O1)OP(=O)(O)OP(=O)(O)O)O)O)OP(=O)(O)O |
physical_description |
Solid |
Origin of Product |
United States |
Retrosynthesis Analysis
AI-Powered Synthesis Planning: Our tool employs the Template_relevance Pistachio, Template_relevance Bkms_metabolic, Template_relevance Pistachio_ringbreaker, Template_relevance Reaxys, Template_relevance Reaxys_biocatalysis model, leveraging a vast database of chemical reactions to predict feasible synthetic routes.
One-Step Synthesis Focus: Specifically designed for one-step synthesis, it provides concise and direct routes for your target compounds, streamlining the synthesis process.
Accurate Predictions: Utilizing the extensive PISTACHIO, BKMS_METABOLIC, PISTACHIO_RINGBREAKER, REAXYS, REAXYS_BIOCATALYSIS database, our tool offers high-accuracy predictions, reflecting the latest in chemical research and data.
Strategy Settings
Precursor scoring | Relevance Heuristic |
---|---|
Min. plausibility | 0.01 |
Model | Template_relevance |
Template Set | Pistachio/Bkms_metabolic/Pistachio_ringbreaker/Reaxys/Reaxys_biocatalysis |
Top-N result to add to graph | 6 |
Feasible Synthetic Routes
Disclaimer and Information on In-Vitro Research Products
Please be aware that all articles and product information presented on BenchChem are intended solely for informational purposes. The products available for purchase on BenchChem are specifically designed for in-vitro studies, which are conducted outside of living organisms. In-vitro studies, derived from the Latin term "in glass," involve experiments performed in controlled laboratory settings using cells or tissues. It is important to note that these products are not categorized as medicines or drugs, and they have not received approval from the FDA for the prevention, treatment, or cure of any medical condition, ailment, or disease. We must emphasize that any form of bodily introduction of these products into humans or animals is strictly prohibited by law. It is essential to adhere to these guidelines to ensure compliance with legal and ethical standards in research and experimentation.