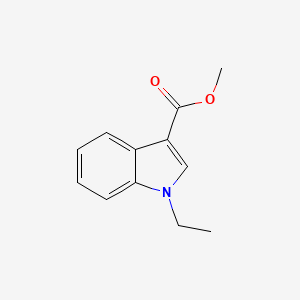
Methyl 1-ethylindole-3-carboxylate
Overview
Description
Methyl 1-ethylindole-3-carboxylate is a heterocyclic organic compound featuring an indole core substituted with an ethyl group at the 1-position and a methyl ester at the 3-position. 1a) have been characterized extensively. For example, crystallographic studies on Methyl 1-methylindole-3-carboxylate reveal a planar indole ring system stabilized by π-π stacking, with hydrogen-bonding interactions involving the ester group contributing to its solid-state stability .
Scientific Research Applications
Biological Applications
Methyl 1-ethylindole-3-carboxylate exhibits a range of biological activities that make it valuable for research and therapeutic development.
Antimicrobial Activity
Research indicates that indole derivatives, including this compound, possess significant antimicrobial properties. They have been shown to inhibit various bacterial strains, making them candidates for developing new antimicrobial agents .
Anticancer Properties
Indole derivatives are being investigated for their potential anticancer effects. Studies suggest that this compound can induce apoptosis in cancer cells by modulating cell cycle regulation and inhibiting pro-inflammatory pathways .
Enzyme Inhibition
This compound has been studied for its ability to inhibit specific enzymes associated with disease processes. For instance, it may act as a nitric oxide synthase inhibitor, which is relevant in cardiovascular diseases .
Therapeutic Potential
The therapeutic potential of this compound extends to several areas:
Pharmaceutical Development
The compound serves as a building block for synthesizing more complex molecules with enhanced biological activity. Its derivatives are being explored for use in developing drugs targeting various diseases, including cancer and infections .
Agrochemical Applications
Given its biological activity, this compound is also being evaluated for applications in agrochemicals, particularly as a potential pesticide or herbicide due to its antimicrobial properties.
Case Studies and Research Findings
Several studies have highlighted the applications of this compound:
Q & A
Basic Research Questions
Q. What are the optimal synthetic routes for Methyl 1-ethylindole-3-carboxylate, and how can purity be validated?
- Methodology : Synthesis typically involves Fischer indole synthesis or alkylation of indole precursors. For example, alkylation at the indole nitrogen with ethyl bromide under basic conditions (e.g., NaH in DMF) can yield the 1-ethyl substituent . Purification via column chromatography (e.g., silica gel, hexane/ethyl acetate gradient) followed by recrystallization improves purity. Validation methods include HPLC (≥98% purity), NMR (to confirm substitution patterns), and UV/Vis spectroscopy (λmax ~280–290 nm, typical for indole derivatives) .
Q. What spectroscopic techniques are critical for characterizing this compound?
- Methodology :
- NMR : H and C NMR confirm substituent positions (e.g., ethyl group at N1, carboxylate at C3). Key signals include the methyl ester (~3.8–4.0 ppm in H NMR) and downfield aromatic protons .
- Mass Spectrometry : High-resolution MS (HRMS) verifies molecular weight (e.g., [M+H]+ at m/z 218.1 for CHNO) .
- X-ray Diffraction : Single-crystal X-ray analysis resolves bond lengths/angles and confirms stereochemistry .
Q. How can researchers troubleshoot low yields in the alkylation step of indole derivatives?
- Methodology : Optimize reaction conditions:
- Use anhydrous solvents (DMF, THF) and inert atmosphere to prevent side reactions.
- Adjust base strength (e.g., NaH vs. KCO) to balance reactivity and selectivity.
- Monitor reaction progress via TLC and quench intermediates to avoid over-alkylation .
Advanced Research Questions
Q. How can density functional theory (DFT) predict the electronic properties of this compound?
- Methodology :
- Use software like Gaussian or ORCA to calculate HOMO/LUMO energies, electrostatic potential maps, and Fukui indices. These predict reactivity sites (e.g., electrophilic substitution at C5 of indole) .
- Compare computed IR/Raman spectra with experimental data to validate models .
- Analyze non-covalent interactions (e.g., π-stacking) using reduced density gradient (RDG) methods .
Q. What strategies resolve contradictions in crystallographic data, such as anomalous bond lengths?
- Methodology :
- Re-examine data collection parameters (e.g., resolution, temperature). High-resolution data (<1.0 Å) reduces errors in bond length determination .
- Use SHELXL’s refinement tools (e.g., restraints for thermal parameters, TWIN/BASF commands for twinned crystals) .
- Validate against databases (e.g., Cambridge Structural Database) to identify outliers .
Q. How are puckering coordinates calculated for non-planar rings in this compound derivatives?
- Methodology :
- Apply the Cremer-Pople method to define a mean plane for the indole ring. Calculate out-of-plane displacements (z) and derive amplitude (q) and phase (φ) parameters .
- Use software like Mercury to visualize puckering and compare with similar structures (e.g., cyclopentane derivatives) .
Q. What experimental and computational approaches assess the bioactivity of this compound analogs?
- Methodology :
- In vitro assays : Test cytotoxicity (MTT assay), enzyme inhibition (e.g., kinase assays), or receptor binding (fluorescence polarization) .
- Molecular docking : Use AutoDock Vina to simulate ligand-receptor interactions (e.g., with cannabinoid receptors, given structural similarity to synthetic cannabinoids) .
- QSAR modeling : Correlate substituent effects (e.g., ethyl vs. pentyl groups) with activity trends .
Q. Notes
Comparison with Similar Compounds
Comparison with Structurally Similar Compounds
Structural and Functional Analogues
Key analogues include:
- Methyl 1-methylindole-3-carboxylate : Shares the indole-3-carboxylate backbone but substitutes a methyl group at the 1-position. Its crystal structure (space group P2₁/c) confirms rigidity due to intermolecular hydrogen bonds involving the ester oxygen .
- Ethyl 1-ethyl-3-methyl-2-oxoindoline-3-carboxylate : An indoline derivative with ethyl and methyl substituents. The saturated six-membered ring and ketone group reduce aromaticity, altering reactivity compared to indole-based esters .
- Methyl 1-methyl-β-carboline-3-carboxylate : A β-carboline derivative with a fused pyrido-indole system. This compound exhibits a higher melting point (>200°C) due to extended conjugation and stronger intermolecular interactions .
- Methyl salicylate : A simpler aromatic ester lacking the indole core. Its volatility and lower molecular weight (152.15 g/mol) contrast sharply with indole derivatives .
Physicochemical Properties
*Estimated properties based on structural analogues.
Preparation Methods
Direct Alkylation-Esterification Sequential Approach
The most widely documented route involves sequential N1-ethylation of indole-3-carboxylic acid followed by esterification. A representative protocol derived from analogous indole alkylation systems involves refluxing indole-3-carboxylic acid with ethyl iodide in methanol using sodium methoxide as a base .
Reaction Mechanism and Conditions
The reaction proceeds via deprotonation of the indole NH by sodium methoxide, forming a resonance-stabilized anion that undergoes nucleophilic attack on ethyl iodide. Subsequent esterification with methanol under acidic conditions yields the target compound. Key parameters include:
-
Molar ratios : A 2:1 excess of ethyl iodide to indole-3-carboxylic acid ensures complete alkylation .
-
Temperature : Reflux conditions (65–70°C) for 4–6 hours optimize both alkylation and esterification .
-
Base selection : Sodium methoxide outperforms weaker bases (e.g., K₂CO₃) by achieving >95% N1-ethylation selectivity .
Typical Yield : 82–89% after recrystallization from methanol-water (3:7) .
One-Pot Alkylation Using Dimethyl Sulfate
Dimethyl sulfate (DMS) serves as a dual alkylating agent for simultaneous N1-ethylation and esterification, though this requires careful stoichiometric control. A modified procedure from indazole methylation systems adapts DMS in methanol with calcium oxide as a base :
Procedure and Optimization
-
Base activation : Calcium oxide (2.0 equiv) is refluxed in methanol to generate calcium methoxide in situ .
-
Alkylation : Indole-3-carboxylic acid (1.0 equiv) is added, followed by dropwise DMS (2.0 equiv) under reflux for 4 hours .
-
Workup : Adjusting the pH to 4 precipitates calcium sulfate, which is filtered, and the filtrate is concentrated to isolate the crude product .
Critical Parameters :
-
DMS addition rate : Slow addition minimizes O-alkylation side products.
-
pH control : Maintaining alkaline conditions (pH >12) during alkylation prevents premature ester hydrolysis .
Yield : 78–85% with HPLC purity >98% after slurry purification .
Transition Metal-Catalyzed Methods
Palladium-catalyzed routes enable modular construction of the indole ring with pre-installed N1-ethyl and C3-ester groups. While specific data for Methyl 1-ethylindole-3-carboxylate is limited, analogous systems using methyl 2-methylindole-3-carboxylate suggest viable pathways:
Intramolecular Cyclization
A reported method employs Pd(OAc)₂ (5 mol%) with XPhos ligand (10 mol%) in DMF at 120°C to cyclize ethyl-substituted enamine precursors.
Key Advantages :
-
Regioselectivity : Directs substitution to the N1 position without protecting groups.
-
Functional group tolerance : Compatible with ester groups at C3.
Challenges :
-
Substrate availability : Requires specialized precursors like ethyl-substituted β-keto esters.
-
Yield : 65–72% in model systems, lower than classical alkylation.
Comparative Analysis of Methods
Method | Yield (%) | Purity (%) | Scalability | Cost Efficiency |
---|---|---|---|---|
Sequential alkylation | 82–89 | 98–99 | High | Moderate |
One-pot DMS | 78–85 | 97–99 | Moderate | High |
Pd-catalyzed | 65–72 | 95–97 | Low | Low |
Key Observations :
-
Classical alkylation methods (Sections 1–2) offer superior yields and scalability for industrial applications.
-
Transition metal catalysis (Section 3) provides synthetic flexibility but remains limited by precursor complexity.
Purification and Analytical Validation
Recrystallization Techniques
Slurry purification in methanol-water (3:7) effectively removes unreacted starting materials and regioisomers (e.g., 2-ethylindole derivatives) .
Spectroscopic Characterization
-
¹H NMR (CDCl₃): δ 1.42 (t, J = 7.1 Hz, 3H, CH₂CH₃), 3.89 (s, 3H, OCH₃), 4.32 (q, J = 7.1 Hz, 2H, NCH₂), 7.20–7.35 (m, 4H, Ar-H) .
-
IR (KBr) : 1715 cm⁻¹ (C=O ester), 1602 cm⁻¹ (C=C aromatic) .
Industrial-Scale Considerations
Large-scale production favors the one-pot DMS method due to reduced solvent usage and shorter reaction times. However, dimethyl sulfate’s toxicity necessitates stringent safety protocols, including closed-system reactors and scrubbers for SO₂ mitigation .
Properties
Molecular Formula |
C12H13NO2 |
---|---|
Molecular Weight |
203.24 g/mol |
IUPAC Name |
methyl 1-ethylindole-3-carboxylate |
InChI |
InChI=1S/C12H13NO2/c1-3-13-8-10(12(14)15-2)9-6-4-5-7-11(9)13/h4-8H,3H2,1-2H3 |
InChI Key |
VVKGPGIOVJYAHI-UHFFFAOYSA-N |
Canonical SMILES |
CCN1C=C(C2=CC=CC=C21)C(=O)OC |
Origin of Product |
United States |
Synthesis routes and methods I
Procedure details
Synthesis routes and methods II
Procedure details
Synthesis routes and methods III
Procedure details
Synthesis routes and methods IV
Procedure details
Disclaimer and Information on In-Vitro Research Products
Please be aware that all articles and product information presented on BenchChem are intended solely for informational purposes. The products available for purchase on BenchChem are specifically designed for in-vitro studies, which are conducted outside of living organisms. In-vitro studies, derived from the Latin term "in glass," involve experiments performed in controlled laboratory settings using cells or tissues. It is important to note that these products are not categorized as medicines or drugs, and they have not received approval from the FDA for the prevention, treatment, or cure of any medical condition, ailment, or disease. We must emphasize that any form of bodily introduction of these products into humans or animals is strictly prohibited by law. It is essential to adhere to these guidelines to ensure compliance with legal and ethical standards in research and experimentation.