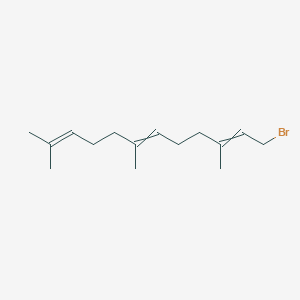
Farnesyl bromide
Overview
Description
Farnesyl bromide, also known as trans,trans-farnesyl bromide, is an organic compound with the molecular formula C15H25Br. It is a brominated derivative of farnesene, a sesquiterpene hydrocarbon. This compound is primarily used as an intermediate in organic synthesis and has applications in various fields, including pharmaceuticals and materials science .
Preparation Methods
Farnesyl bromide can be synthesized through several methods. One common synthetic route involves the bromination of farnesol. The process typically includes the following steps :
Starting Material: Farnesol is used as the starting material.
Bromination: The bromination reaction is carried out using phosphorus tribromide (PBr3) in an inert solvent such as diethyl ether. The reaction is conducted at low temperatures (0°C) to control the reaction rate and prevent side reactions.
Workup: The reaction mixture is quenched with water, and the organic layer is separated. The product is purified by extraction with hexane and drying over anhydrous sodium sulfate.
Chemical Reactions Analysis
Farnesyl bromide undergoes various chemical reactions, including:
Scientific Research Applications
Farnesyl bromide has several scientific research applications:
Organic Synthesis: It is used as an intermediate in the synthesis of various organic compounds, including pharmaceuticals and agrochemicals.
Medicinal Chemistry: The compound is employed in the synthesis of vitamin derivatives and other bioactive molecules.
Materials Science: It is used in the preparation of advanced materials, such as polymers and coatings.
Mechanism of Action
The mechanism of action of Farnesyl bromide involves its reactivity as a brominated compound. The bromine atom can act as a leaving group in substitution reactions, allowing the compound to participate in various chemical transformations. The molecular targets and pathways involved depend on the specific reactions and applications .
Comparison with Similar Compounds
Farnesyl bromide can be compared with other similar compounds, such as:
This compound: Similar in structure but with different stereochemistry.
Farnesyl Alcohol: The alcohol derivative of farnesene, used in different applications.
Farnesyl Acetate: An ester derivative with distinct properties and uses.
These compounds share similar structural features but differ in their functional groups and reactivity, making each unique for specific applications.
Properties
Molecular Formula |
C15H25Br |
---|---|
Molecular Weight |
285.26 g/mol |
IUPAC Name |
1-bromo-3,7,11-trimethyldodeca-2,6,10-triene |
InChI |
InChI=1S/C15H25Br/c1-13(2)7-5-8-14(3)9-6-10-15(4)11-12-16/h7,9,11H,5-6,8,10,12H2,1-4H3 |
InChI Key |
FOFMBFMTJFSEEY-UHFFFAOYSA-N |
Canonical SMILES |
CC(=CCCC(=CCCC(=CCBr)C)C)C |
Origin of Product |
United States |
Synthesis routes and methods I
Procedure details
Synthesis routes and methods II
Procedure details
Synthesis routes and methods III
Procedure details
Disclaimer and Information on In-Vitro Research Products
Please be aware that all articles and product information presented on BenchChem are intended solely for informational purposes. The products available for purchase on BenchChem are specifically designed for in-vitro studies, which are conducted outside of living organisms. In-vitro studies, derived from the Latin term "in glass," involve experiments performed in controlled laboratory settings using cells or tissues. It is important to note that these products are not categorized as medicines or drugs, and they have not received approval from the FDA for the prevention, treatment, or cure of any medical condition, ailment, or disease. We must emphasize that any form of bodily introduction of these products into humans or animals is strictly prohibited by law. It is essential to adhere to these guidelines to ensure compliance with legal and ethical standards in research and experimentation.