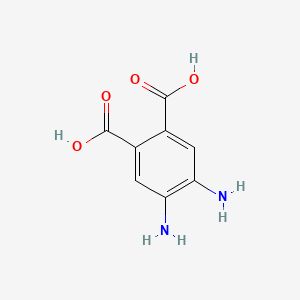
4,5-Diaminophthalic acid
- Click on QUICK INQUIRY to receive a quote from our team of experts.
- With the quality product at a COMPETITIVE price, you can focus more on your research.
Overview
Description
Phthalic acid derivatives feature a benzene ring with two carboxylic acid groups. In 4,5-diaminophthalic acid, amino (-NH₂) groups replace hydrogen atoms at the 4 and 5 positions (ortho to the carboxylic acids). This structural motif likely confers unique reactivity, solubility, and coordination properties, making it valuable for applications in pharmaceuticals, agrochemicals, and materials science.
Chemical Reactions Analysis
Decarboxylation Reactions
4,5-Diaminophthalic acid undergoes thermal or microbial decarboxylation to form substituted benzoic acid derivatives. For example:
-
Microbial decarboxylation by Pseudomonas testosteroni or Bacillus spp. converts 4,5-dihydroxyphthalic acid (structurally analogous to the diamino derivative) to protocatechuic acid (3,4-dihydroxybenzoic acid) . Similarly, halogenated or aminated phthalates are decarboxylated to corresponding benzoic acids (e.g., 3-chlorobenzoic acid from 4-chlorophthalic acid) .
-
Thermal decarboxylation in polar solvents (e.g., water or alkanols) at 100–170°C removes one or both carboxylic acid groups, yielding intermediates like 4,5-diaminobenzoic acid .
Table 1: Decarboxylation Pathways
Substrate | Conditions | Product | Reference |
---|---|---|---|
This compound | Bacillus spp., aerobic | 3,4-Diaminobenzoic acid | |
This compound | Heating in alkanol (150°C) | 4,5-Diaminobenzoic acid |
Condensation Reactions
The amino and carboxylic acid groups enable condensation with nucleophiles:
-
Amide/imide formation : Reaction with amines (e.g., ethylenediamine) in aprotic solvents (DMF, acetic acid) forms cyclic imides or bis-amide derivatives. For example, phthalic anhydride reacts with ethylenediamine to yield N,N'-diphthaloyl-1,2-ethylenediamine . Similar pathways apply to this compound, producing polycyclic amides .
-
Schiff base synthesis : The amino groups condense with aldehydes (e.g., formaldehyde) to form Schiff bases, which are precursors for coordination complexes .
Table 2: Condensation Products
Reactant | Conditions | Product | Reference |
---|---|---|---|
Ethylenediamine | Acetic acid, reflux | Bis-amide derivative | |
Aldehydes | Methanol, RT | Schiff base complexes |
Coordination Chemistry
The amino and carboxylic acid groups act as polydentate ligands for metal ions:
-
Transition metal complexes : Forms stable complexes with Co(II), Ni(II), Cu(II), and Zn(II) in 1:1 or 1:2 molar ratios. These complexes exhibit antimicrobial activity .
-
Catalytic applications : Copper phthalocyanine derivatives (synthesized from phthalic anhydride analogs) serve as pigments or catalysts in oxidation reactions .
Table 3: Metal Complex Properties
Metal Ion | Ligand Ratio | Application | Reference |
---|---|---|---|
Cu(II) | 1:1 | Antimicrobial agent | |
Co(II) | 1:2 | Dye intermediate |
Functionalization via Nitrosation and Diazotization
The amino groups participate in nitrosation and diazotization:
Q & A
Basic Research Questions
Q. What are the standard synthetic routes for preparing 4,5-diaminophthalic acid, and how do reaction conditions influence yield?
- Methodological Answer : The synthesis typically involves the reduction of nitro groups in precursors like 4,5-dinitrophthalic acid using catalytic hydrogenation (e.g., Pd/C or Raney Ni) or chemical reductants (e.g., Sn/HCl). Yield optimization requires precise control of pH, temperature, and reaction time. For example, hydrogenation at 60–80°C under 3–5 bar H₂ pressure achieves >80% yield, but over-reduction must be avoided by monitoring reaction progress via TLC or HPLC .
Q. Which spectroscopic techniques are most effective for characterizing this compound?
- Methodological Answer :
- NMR : ¹H and ¹³C NMR confirm amine and carboxylic acid proton environments. Aromatic protons appear as doublets (δ 6.8–7.2 ppm), while NH₂ groups show broad signals (δ 4.5–5.5 ppm) .
- FTIR : Peaks at ~3350 cm⁻¹ (N-H stretch), 1680 cm⁻¹ (C=O), and 1600 cm⁻¹ (aromatic C=C) validate functional groups.
- Mass Spectrometry : ESI-MS in negative mode typically displays [M−H]⁻ ions at m/z 208.
Q. How does pH affect the stability of this compound in aqueous solutions?
- Methodological Answer : Stability studies using UV-Vis spectroscopy show maximal stability at pH 4–5. Outside this range, degradation occurs via hydrolysis of amine or carboxylic acid groups. Buffered solutions (e.g., phosphate or acetate) are recommended for long-term storage. Kinetic analysis at varying pH levels (2–10) reveals a degradation half-life of >48 hours within the optimal range .
Q. What are the key solubility properties of this compound in common solvents?
- Methodological Answer : The compound is sparingly soluble in water (0.2 g/L at 25°C) but dissolves in polar aprotic solvents (e.g., DMF, DMSO). Solubility can be enhanced via salt formation (e.g., sodium or ammonium salts). A phase diagram study in ethanol/water mixtures shows solubility peaks at 70% ethanol .
Advanced Research Questions
Q. How can regioselectivity challenges during functionalization of this compound be addressed?
- Methodological Answer : Steric and electronic effects dictate reactivity. For example, acylation of amine groups requires protecting carboxylic acids (e.g., esterification with MeOH/H₂SO₄). DFT calculations (B3LYP/6-31G*) predict higher electron density at the para-amine, guiding selective modifications. Experimental validation via X-ray crystallography confirms regioselectivity in derivatives .
Q. What strategies resolve contradictions between experimental data and computational models for this compound complexes?
- Methodological Answer : Cross-validate spectroscopic data (e.g., UV-Vis, EPR) with DFT-optimized geometries (e.g., bond lengths, angles). For metal complexes, compare experimental magnetic moments with spin-state predictions. Discrepancies often arise from solvation effects—incorporate implicit solvent models (e.g., PCM) in simulations .
Q. How do metal coordination modes of this compound influence catalytic or electronic properties?
- Methodological Answer : Study coordination via X-ray diffraction and cyclic voltammetry. For example, Cu(II) complexes exhibit square-planar geometry (confirmed by XRD), with redox activity at E₁/₂ = −0.25 V (vs. Ag/AgCl). Catalytic efficiency in oxidation reactions correlates with ligand-to-metal charge transfer bands observed at 450–500 nm .
Q. What advanced chromatographic methods separate isomers or degradation products of this compound?
- Methodological Answer : Use chiral HPLC (e.g., Chiralpak IA column) with a mobile phase of hexane:isopropanol (90:10) + 0.1% TFA. For degradation products, LC-MS/MS with a C18 column (gradient: 5–95% acetonitrile in 0.1% formic acid) identifies hydrolyzed intermediates .
Q. How can computational modeling predict the reactivity of this compound in novel reactions?
- Methodological Answer : Perform frontier molecular orbital (FMO) analysis using Gaussian09. The HOMO (-5.2 eV) localizes on the diamine moiety, indicating nucleophilic sites. Transition state modeling (TS Berny algorithm) predicts activation energies for reactions like Michael additions .
Q. What are the ethical considerations in reporting contradictory data for this compound studies?
- Methodological Answer : Disclose all raw data (e.g., via supplementary materials) and perform sensitivity analyses to identify outliers. Use consensus frameworks like COPE guidelines to address authorship disputes or reproducibility issues. Transparently document instrument calibration and statistical methods (e.g., ANOVA for batch variations) .
Comparison with Similar Compounds
Comparison with Structurally Similar Compounds
4,5-Difluorophthalic Acid ()
- Structure: Fluorine atoms at positions 4 and 5 instead of amino groups.
- Applications :
- Key Differences: Fluorine’s electron-withdrawing nature reduces nucleophilicity compared to amino groups. amino-functionalized analogs.
3,4-Diaminobenzoic Acid ()
- Structure: A benzoic acid derivative with amino groups at positions 3 and 3.
- Applications: Pharmacology: Used in reference standards and intermediate synthesis (similar to ’s caffeic acid). Coordination Chemistry: Amino and carboxylic acid groups enable metal-organic framework (MOF) formation.
- Key Differences: Single carboxylic acid group vs. two in phthalic acid derivatives, altering acidity and solubility. Amino groups at adjacent positions (3,4) may sterically hinder interactions compared to 4,5-substitution.
Caffeic Acid and Chlorogenic Acid (CGA) ()
- Structure: Phenolic derivatives with hydroxyl (-OH) and carboxylic acid groups.
- Mechanisms :
- Key Differences: Hydroxyl groups lack the basicity and coordination versatility of amino groups. CGA’s quinic acid moiety enhances solubility but reduces structural rigidity compared to planar phthalic acid derivatives.
4,5-Diaryl Thiophene-2-Carboxylic Acid Derivatives ()
- Structure : Thiophene ring with aryl substituents and a carboxylic acid group.
- Applications :
- Key Differences: Sulfur in the thiophene ring alters electronic properties (e.g., increased π-acidity) vs. benzene-based phthalic acids.
Comparative Data Table
Compound | Functional Groups | Key Applications | Biological Activity | Unique Properties |
---|---|---|---|---|
4,5-Diaminophthalic Acid | 2 × -NH₂, 2 × -COOH | Hypothetical: MOFs, drug intermediates | Potential antioxidant/chelator | High coordination versatility |
4,5-Difluorophthalic Acid | 2 × -F, 2 × -COOH | Pharmaceuticals, agrochemicals | N/A (precursor role) | Metabolic stability |
3,4-Diaminobenzoic Acid | 2 × -NH₂, 1 × -COOH | MOFs, reference standards | Limited data | Steric hindrance at 3,4 |
Caffeic Acid | 2 × -OH, 1 × -COOH | Neuroprotection, antioxidants | ROS scavenging, anti-apoptotic | Phenolic redox activity |
Thiophene-2-carboxylic Acid Derivatives | 1 × -COOH, aryl groups | Antimicrobial, anticancer | Gram-positive inhibition | Thiophene π-acidity |
Research Findings and Mechanistic Insights
- Antioxidant Potential: Amino groups in this compound may enhance metal chelation (e.g., Fe²⁺/Cu²⁺) and radical scavenging compared to hydroxyl or fluorine substituents, as seen in CGA and 4,5-difluorophthalic acid .
- Coordination Chemistry: Dual -COOH and -NH₂ groups could enable novel MOFs with tunable porosity, similar to ’s coordination polymers .
- Biological Activity: Structural analogs like thiophene-2-carboxylic acid derivatives show that electron-withdrawing groups (e.g., -F, -COOH) enhance antimicrobial efficacy, suggesting this compound might exhibit similar or superior activity .
Properties
Molecular Formula |
C8H8N2O4 |
---|---|
Molecular Weight |
196.16 g/mol |
IUPAC Name |
4,5-diaminophthalic acid |
InChI |
InChI=1S/C8H8N2O4/c9-5-1-3(7(11)12)4(8(13)14)2-6(5)10/h1-2H,9-10H2,(H,11,12)(H,13,14) |
InChI Key |
XOFYHIQHLWORCL-UHFFFAOYSA-N |
Canonical SMILES |
C1=C(C(=CC(=C1N)N)C(=O)O)C(=O)O |
Origin of Product |
United States |
Disclaimer and Information on In-Vitro Research Products
Please be aware that all articles and product information presented on BenchChem are intended solely for informational purposes. The products available for purchase on BenchChem are specifically designed for in-vitro studies, which are conducted outside of living organisms. In-vitro studies, derived from the Latin term "in glass," involve experiments performed in controlled laboratory settings using cells or tissues. It is important to note that these products are not categorized as medicines or drugs, and they have not received approval from the FDA for the prevention, treatment, or cure of any medical condition, ailment, or disease. We must emphasize that any form of bodily introduction of these products into humans or animals is strictly prohibited by law. It is essential to adhere to these guidelines to ensure compliance with legal and ethical standards in research and experimentation.