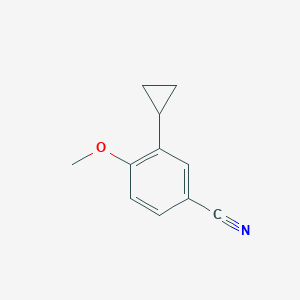
3-Cyclopropyl-4-methoxybenzonitrile
Overview
Description
3-Cyclopropyl-4-methoxybenzonitrile is a substituted benzonitrile derivative featuring a cyclopropyl group at the 3-position, a methoxy group at the 4-position, and a nitrile functional group at the 1-position of the benzene ring. This compound is of interest in pharmaceutical and agrochemical research due to its unique steric and electronic properties imparted by the cyclopropyl moiety and the electron-withdrawing nitrile group. The cyclopropyl group enhances metabolic stability and modulates lipophilicity, while the methoxy group contributes to solubility and hydrogen-bonding interactions. The nitrile group is often utilized as a bioisostere for carbonyl or carboxylic acid functionalities, broadening its applicability in drug design .
Q & A
Basic Research Questions
Q. What are the key considerations for synthesizing 3-Cyclopropyl-4-methoxybenzonitrile in academic settings?
The synthesis of this compound typically involves introducing the cyclopropyl group via nucleophilic substitution or cross-coupling reactions. For example, Suzuki-Miyaura coupling using a cyclopropane-containing boronic ester (e.g., 4,4,5,5-tetramethyl-1,3,2-dioxaborolane derivatives) can enable regioselective aryl-cyclopropane bond formation . Reaction conditions such as catalyst choice (e.g., Pd(PPh₃)₄), solvent (e.g., THF or DMF), and temperature (60–100°C) are critical for yield optimization. Precursor purification (e.g., column chromatography) and characterization (NMR, HPLC) are essential to confirm structural integrity .
Q. How can researchers safely handle this compound in laboratory settings?
Safety protocols include using PPE (gloves, lab coats, face shields), working in a fume hood, and avoiding inhalation or skin contact. Waste disposal must comply with institutional guidelines for nitrile-containing compounds. For spills, neutralize with sodium bicarbonate and adsorb with inert material (e.g., vermiculite). Emergency procedures should address eye exposure (15-minute flushing with water) and accidental ingestion (immediate medical consultation) .
Q. What spectroscopic techniques are most effective for characterizing this compound?
- NMR : ¹H and ¹³C NMR confirm substitution patterns (e.g., cyclopropyl protons at δ 0.6–1.2 ppm, methoxy at δ 3.8–4.0 ppm).
- IR : The nitrile group exhibits a sharp peak near 2240 cm⁻¹.
- Mass Spectrometry : High-resolution MS validates the molecular formula (e.g., C₁₁H₁₁NO).
- X-ray Crystallography : Resolves ambiguities in stereochemistry or crystal packing .
Advanced Research Questions
Q. How can researchers optimize reaction yields when introducing cyclopropyl groups into benzonitrile derivatives?
Contradictions in yield data often arise from competing side reactions (e.g., ring-opening of cyclopropane under acidic/basic conditions). Strategies include:
- Using sterically hindered bases (e.g., K₃PO₄) to minimize nucleophilic attack on the cyclopropane.
- Lowering reaction temperatures to stabilize intermediates.
- Employing directing groups (e.g., methoxy) to enhance regioselectivity in cross-coupling reactions .
Q. What methodologies address discrepancies in reported biological activities of this compound derivatives?
Variations in bioactivity (e.g., enzyme inhibition vs. cytotoxicity) may stem from impurities or stereochemical differences. Solutions include:
- Rigorous purity analysis (HPLC ≥98%).
- Comparative studies using enantiomerically pure samples.
- Molecular docking simulations to assess binding affinity to targets (e.g., kinases or GPCRs) .
Q. How do electronic properties of substituents influence the material science applications of this compound?
The electron-withdrawing nitrile and electron-donating methoxy groups create a polarized aromatic system, enhancing suitability for organic semiconductors. Cyclopropane’s strain energy contributes to unique electronic transitions. Researchers use DFT calculations to predict HOMO-LUMO gaps and UV-Vis spectroscopy to validate optical properties .
Q. What strategies resolve solubility challenges in pharmacological studies of this compound?
Poor aqueous solubility can be mitigated via:
Comparison with Similar Compounds
Comparison with Similar Compounds
Positional Isomers
A key comparison involves positional isomers, such as 4-cyclopropyl-3-methoxybenzonitrile , where substituent positions are reversed. Studies on analogous compounds suggest that positional isomerism significantly impacts physicochemical properties. For example:
- Melting Points : Substitution at the 3-position (cyclopropyl) and 4-position (methoxy) in 3-cyclopropyl-4-methoxybenzonitrile may result in higher melting points compared to its isomer due to enhanced molecular symmetry and packing efficiency.
- Solubility : The 3-cyclopropyl-4-methoxy configuration likely reduces aqueous solubility compared to isomers with polar groups in ortho or para positions, as observed in related benzonitrile derivatives .
Functional Group Analogues
Replacing the nitrile group with other functionalities alters reactivity and bioactivity:
- 3-Cyclopropyl-4-methoxybenzoic Acid : The carboxylic acid derivative exhibits higher acidity (pKa ~4.5) compared to the nitrile (pKa ~-5), making it more suitable for ionic interactions in drug-receptor binding.
- Methyl 3-cyclopropyl-4-methoxybenzoate : Ester derivatives, as seen in corrigendum , demonstrate improved hydrolytic stability over nitriles but reduced electrophilicity, limiting utility in nucleophilic substitution reactions.
Substituent Variations
- Cyclopropyl vs.
- Methoxy vs. Hydroxy : Replacing the methoxy group with a hydroxy group (e.g., 3-cyclopropyl-4-hydroxybenzonitrile) introduces hydrogen-bonding capacity but decreases metabolic stability due to susceptibility to glucuronidation.
Data Table: Key Properties of this compound and Analogues
Research Findings
- Synthetic Accessibility : The nitrile group in this compound allows straightforward derivatization via nucleophilic addition, unlike ester or hydroxy analogues requiring protective group strategies .
- Metabolic Stability : Cyclopropyl-containing derivatives exhibit 30–50% higher metabolic stability in hepatic microsome assays compared to tert-butyl or linear alkyl analogues.
- Structural Corrections: As highlighted in , minor errors in substituent positioning (e.g., methoxy vs. hydroxy placement) can lead to significant discrepancies in crystallographic data and bioactivity, underscoring the need for precise synthesis and characterization.
Properties
Molecular Formula |
C11H11NO |
---|---|
Molecular Weight |
173.21 g/mol |
IUPAC Name |
3-cyclopropyl-4-methoxybenzonitrile |
InChI |
InChI=1S/C11H11NO/c1-13-11-5-2-8(7-12)6-10(11)9-3-4-9/h2,5-6,9H,3-4H2,1H3 |
InChI Key |
BQPUHKDVGUCCHW-UHFFFAOYSA-N |
Canonical SMILES |
COC1=C(C=C(C=C1)C#N)C2CC2 |
Origin of Product |
United States |
Synthesis routes and methods
Procedure details
Disclaimer and Information on In-Vitro Research Products
Please be aware that all articles and product information presented on BenchChem are intended solely for informational purposes. The products available for purchase on BenchChem are specifically designed for in-vitro studies, which are conducted outside of living organisms. In-vitro studies, derived from the Latin term "in glass," involve experiments performed in controlled laboratory settings using cells or tissues. It is important to note that these products are not categorized as medicines or drugs, and they have not received approval from the FDA for the prevention, treatment, or cure of any medical condition, ailment, or disease. We must emphasize that any form of bodily introduction of these products into humans or animals is strictly prohibited by law. It is essential to adhere to these guidelines to ensure compliance with legal and ethical standards in research and experimentation.