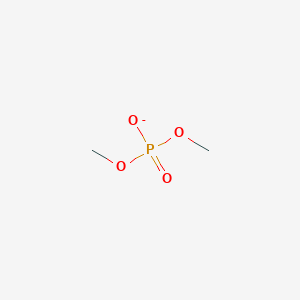
Dimethyl phosphate anion
Overview
Description
The dimethyl phosphate anion (DMP), with the chemical formula (CH₃O)₂PO₂⁻, is a fundamental model compound for studying the phosphodiester backbone of nucleic acids (DNA and RNA) . Its simplified structure mimics the repeating phosphate-sugar backbone in nucleic acids, making it critical for understanding vibrational modes, conformational flexibility, and interactions with metal ions or solvents. DMP exists in gauche-gauche (gg), gauche-trans (gt), and trans-trans (tt) conformations, with the gg conformation being the most stable in aqueous solutions due to favorable hydration effects .
DMP is also widely employed in ionic liquids (ILs) for applications such as biomass pretreatment and absorption refrigeration, owing to its strong hydrogen-bonding capacity and tunable physicochemical properties .
Scientific Research Applications
Ionic Liquids and Petrochemical Applications
Ionic liquids (ILs) containing dimethyl phosphate anion have emerged as efficient materials in petrochemical processes. These ILs are characterized by their low volatility and ability to dissolve a wide range of substances, making them suitable for tasks such as:
- Desulfurization of Fuels : ILs-DMP have shown promise in the desulfurization of diesel and fuel oils, enhancing the removal of sulfur compounds. A study indicated that the DMP anion increases the hydrophilicity of ILs, facilitating better extraction processes compared to traditional solvents .
- Separation Processes : Research has demonstrated that ILs-DMP can effectively separate asphaltenes from crude oil, utilizing their unique solvation properties .
Biopolymer Dissolution
The ability of this compound to dissolve biopolymers is noteworthy, particularly in cellulose dissolution. The following points highlight its significance:
- Cellulose Solubility : DMP is recognized as a strong hydrogen bond acceptor, which enhances its capacity to dissolve cellulose. Studies have shown that certain ILs with DMP can completely convert cellulose into soluble sugars, indicating potential applications in biofuel production .
- Food Processing Byproducts : DMP-containing ionic liquids have been successfully employed to dissolve agricultural waste products like corncobs, showcasing their utility in sustainable practices .
Ion-Pairing and Nucleic Acid Research
This compound serves as a model compound for studying interactions within nucleic acids:
- Ion-Pair Formation : Research indicates that DMP interacts with metal cations (e.g., Na, Mg, Ca) in aqueous solutions, forming ion pairs that are critical for understanding nucleic acid stability and interactions . The formation constants for these ion pairs provide insights into binding mechanisms relevant to RNA structures.
- Spectroscopic Studies : Techniques such as nuclear magnetic resonance spectroscopy have been utilized to explore the hydration dynamics and ion association of DMP with various cations, contributing valuable data for biochemical applications .
Computational Chemistry
Computational studies involving this compound have contributed to our understanding of its structural properties and interactions:
- Molecular Simulations : DMP has been used in simulations to mimic the phosphate group in nucleic acids, aiding in the exploration of steric and electronic interactions that influence biochemical reactions .
- Hydration Studies : Various models have been applied to investigate the hydration behavior of DMP, revealing important characteristics about solute-solvent interactions that are applicable in biochemical contexts .
Industrial Applications
Beyond research, this compound finds practical applications in various industries:
- Lubricants : ILs-DMP are being explored as high-temperature lubricants due to their thermal stability and low volatility, which can enhance machinery performance under extreme conditions .
- Solvents : The unique properties of DMP-containing ionic liquids make them suitable solvents for gas chromatography and other analytical techniques, improving detection limits and resolution .
Case Studies and Data Tables
Chemical Reactions Analysis
Hydrolysis Pathways and Mechanisms
DMP⁻ undergoes hydrolysis via distinct mechanisms depending on pH and solvation:
Acidic and Neutral Conditions
-
Monoanion hydrolysis proceeds through a dissociative mechanism involving protonation of the leaving group in the transition state (TS). The measured Brønsted coefficient (βₗ₋) of −0.27 supports TS stabilization via partial bond cleavage .
-
Activation parameters : ΔH‡ = 30.0 kcal/mol; ΔS‡ = −0.6 kcal/mol·K .
Alkaline Conditions
-
Dianion hydrolysis occurs via a concerted, dissociative TS with minimal nucleophilic participation. Internal electron donation from non-bridging oxygens weakens the P–O bond, accelerating hydrolysis (rate = 7 × 10⁻¹⁶ s⁻¹ at 25°C for analogous diesters) .
-
Kinetic data :
Nucleophilic Reactions with Elemental Sulfur
DMP⁻ reacts with S₈ via nucleophilic attack at the anionic oxygen atom, leading to S₈ ring opening and formation of sulfurized products:
-
Mechanism : DFT calculations (B3LYP/STO-3G) confirm a low-energy pathway involving sulfur insertion into the P–O bond (ΔE ≈ −207.3 kcal/mol) .
-
Reactivity trend : DMP⁻ > tri-n-butylmethylphosphonium dimethylphosphate due to charge delocalization in the imidazolium counterion .
Gas-Phase Reactivity
In the gas phase, DMP⁻ exhibits ambident nucleophilicity and forms stable complexes:
-
Generation : Produced via proton abstraction by phenyl nitrene anion radical (PhN⁻) .
-
Reactions with methyl halides :
-
Thermochemical properties :
Ion-Pairing Effects in Solution
Metal cations modulate DMP⁻ reactivity through ion-pair formation:
-
Stability constants (log Kₐ) :
-
Dielectric relaxation spectroscopy reveals Mg²⁺-DMP⁻ complexes have lifetimes (~10 ps) comparable to rotational relaxation times .
Solvent and Electronic Polarization Effects
Ab initio MD simulations (Car–Parrinello) show:
Q & A
Q. Basic: What are the common laboratory synthesis methods for dimethyl phosphate anion?
Methodological Answer:
this compound can be synthesized via phosphoramidite chemistry. For example, bis(N,N-diisopropyl amino)methoxyphosphine is prepared by reacting phosphorus trichloride with methanol and N,N-diisopropylamine under controlled conditions . Alternatively, reaction of dimethyl(phenylsulfonyl)amidophosphate with tetramethylammonium hydroxide in isopropanol yields crystalline salts, as demonstrated by X-ray diffraction studies . Key steps include distillation to isolate intermediates and strict temperature control to prevent side reactions.
Q. Basic: Which analytical techniques are effective for detecting this compound in environmental or biological samples?
Methodological Answer:
- Ion Chromatography (IC): Use ammonium phosphate buffer (pH 7.5) with acetonitrile-methanol mobile phases for separation, followed by conductivity detection .
- Spectrophotometry: React with molybdenum blue reagent under acidic conditions; measure absorbance at 880 nm after ascorbic acid reduction .
- Vibrational Spectroscopy: Assign conformation-sensitive bands (e.g., PO₂⁻ symmetric/asymmetric stretches at ~1100 cm⁻¹) via Raman or FTIR, supported by DFT-optimized geometries .
Comparison with Similar Compounds
Organophosphate Anions
Arsenate (AsO₄³⁻)
Arsenate, a toxic analog of phosphate, shares structural and electronic similarities with DMP. Both exhibit tetrahedral geometry and comparable pKa values (AsO(OH)₃: 2.26, 6.76, 11.29; PO(OH)₃: 2.15, 7.20, 12.35). Arsenate competes with phosphate in biological systems, hijacking transporters like zebrafish NaPi-IIb1 (Km = 0.22 mM for AsO₄³⁻ vs. 0.05 mM for PO₄³⁻) . This competitive inhibition underscores the specificity of phosphate-binding proteins.
Sulfonamide Phosphate Derivatives
Compounds like dimethyl(phenylsulfonylamido)phosphate (CH₃)₂PO₂⁻(SO₂C₆H₅N⁻) exhibit distorted tetrahedral geometry at phosphorus (P–O = 1.46 Å, P–N = 1.59 Å) . Unlike DMP, these derivatives lack direct metal coordination in crystal structures, relying instead on ionic interactions (e.g., with tetramethylammonium cations) . Their rigid sulfonamide groups limit conformational flexibility compared to DMP.
Metal Ion Interactions
DMP shows distinct binding preferences for metal ions, critical in nucleic acid stability:
The Irving-Williams order (Mn²⁺ < Fe²⁺ < Co²⁺ < Ni²⁺ < Cu²⁺ > Zn²⁺) aligns with DMP’s binding affinity trends .
Key Research Findings
- Vibrational Spectroscopy : DMP’s PO₂⁻ stretching modes are sensitive to conformation (gg vs. gt/tt), providing insights into nucleic acid dynamics .
- Environmental Impact: Organophosphates like diethyl phosphate show paradoxical associations with reduced ASD risk (β = -0.49, 95% CI: -0.85 to -0.13), though mechanisms remain unclear .
Preparation Methods
Industrial Synthesis via Phosphorus Trichloride Esterification
The most widely implemented industrial method for DMP⁻ production involves the reaction of phosphorus trichloride (PCl₃) with methanol (CH₃OH) under controlled conditions . This solventless liquid-phase process optimizes yield while minimizing environmental impact through closed-loop by-product recovery.
Reaction Mechanism and Stoichiometry
The esterification occurs in four stages:
-
Esterification : PCl₃ reacts with methanol at −10°C to −20°C under vacuum (−90 kPa), producing dimethylphosphite intermediate and gaseous HCl/CH₃Cl .
-
Deacidification : Residual HCl is removed at 75–80°C, yielding purified dimethylphosphite .
-
Fore-running : Volatile impurities (methanol, trimethyl phosphite) are distilled at 80–85°C .
-
Rectification : High-purity dimethylphosphite (≥98.9%) is obtained by vacuum distillation at 122–124°C .
Critical Process Parameters
Parameter | Optimal Range | Impact on Yield |
---|---|---|
PCl₃:MeOH Molar Ratio | 1.0–1.2:3 | ↑ Ratio → ↑ Purity |
Esterification Temp | −10°C to −20°C | Prevents side reactions |
Vacuum Pressure | ≤−90 kPa | Accelerates HCl removal |
Rectification Time | 50–55 sec | Minimizes thermal degradation |
This method achieves 86–93% yield, with HCl and CH₃Cl recovered as saleable by-products .
Laboratory-Scale Preparation via Sodium Dimethyl Phosphate
Sodium dimethyl phosphate (NaDMP) serves as a precursor for DMP⁻ generation through dissociation in aqueous solutions . Commercial NaDMP (93% purity) undergoes purification via recrystallization before use.
Dissociation Dynamics
In water, NaDMP dissociates into Na⁺ and DMP⁻ ions:
Dielectric relaxation spectroscopy confirms ion-pairing equilibria with Mg²⁺, Ca²⁺, and Na⁺, where DMP⁻ forms contact ion pairs (CIPs) with divalent cations .
Purity Optimization
-
Fore-running : Removes volatile contaminants (e.g., ethyl methyl phosphate) at 80°C under vacuum .
-
Crystallization : Ethanol/water mixtures (3:1 v/v) yield NaDMP with ≤0.5% impurities .
Ionic Liquid Synthesis Incorporating DMP⁻
DMP⁻-based ionic liquids (ILs) are synthesized via quaternization of amines or phosphines with dimethyl phosphate esters . Two principal routes exist:
Direct Alkylation of Amines
Triethylamine reacts with trimethyl phosphate under reflux:
2\text{H}5\text{)}3 + \text{(CH}3\text{O)}3\text{PO} \rightarrow \text{[N(C}2\text{H}5\text{)}3\text{H]}^+\text{DMP}^- + 2\text{CH}_3\text{OH}
Yields exceed 85% when conducted in acetonitrile at 60°C for 12 h .
Anion Exchange from Chloride Salts
Pre-formed ILs (e.g., 1-butyl-3-methylimidazolium chloride) undergo metathesis with NaDMP:
This solvent-free method achieves >90% conversion at 25°C within 2 h .
Acid-Base Deprotonation of Dimethyl Phosphate
DMP⁻ is accessible via deprotonation of dimethyl phosphoric acid using strong bases (NaOH, KOH) :
3\text{O)}2\text{POOH} + \text{NaOH} \rightarrow \text{(CH}3\text{O)}2\text{POO}^-\text{Na}^+ + \text{H}_2\text{O}
The pKa of dimethyl phosphoric acid is 1.1, enabling quantitative deprotonation at pH >3 .
Comparative Analysis of Preparation Methods
Method | Yield (%) | Purity (%) | Scalability | By-Product Handling |
---|---|---|---|---|
PCl₃ Esterification | 86–93 | 98.9 | Industrial | HCl/CH₃Cl recovery |
NaDMP Dissociation | 95–98 | 99.5 | Laboratory | Ethanol recycling |
IL Alkylation | 85–90 | 99.0 | Pilot-scale | Methanol recovery |
Acid-Base Reaction | 100 | 97.0 | Bench-scale | Aqueous waste |
Properties
CAS No. |
7351-83-9 |
---|---|
Molecular Formula |
C2H6O4P- |
Molecular Weight |
125.04 g/mol |
IUPAC Name |
dimethyl phosphate |
InChI |
InChI=1S/C2H7O4P/c1-5-7(3,4)6-2/h1-2H3,(H,3,4)/p-1 |
InChI Key |
KKUKTXOBAWVSHC-UHFFFAOYSA-M |
Canonical SMILES |
COP(=O)([O-])OC |
Origin of Product |
United States |
Synthesis routes and methods
Procedure details
Disclaimer and Information on In-Vitro Research Products
Please be aware that all articles and product information presented on BenchChem are intended solely for informational purposes. The products available for purchase on BenchChem are specifically designed for in-vitro studies, which are conducted outside of living organisms. In-vitro studies, derived from the Latin term "in glass," involve experiments performed in controlled laboratory settings using cells or tissues. It is important to note that these products are not categorized as medicines or drugs, and they have not received approval from the FDA for the prevention, treatment, or cure of any medical condition, ailment, or disease. We must emphasize that any form of bodily introduction of these products into humans or animals is strictly prohibited by law. It is essential to adhere to these guidelines to ensure compliance with legal and ethical standards in research and experimentation.