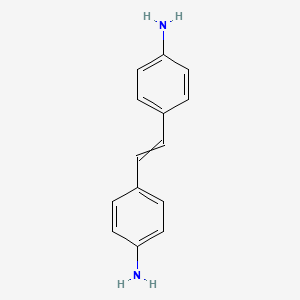
4,4'-Diaminostilbene
Overview
Description
4,4'-Diaminostilbene-2,2'-disulfonic acid (DASD, also termed DSD acid) is a sulfonated aromatic diamine with the molecular formula C₁₄H₁₄N₂O₆S₂. It is synthesized via catalytic hydrogenation of 4,4’-dinitrostilbene-2,2’-disulfonic acid using Pd-Cu/sibunite catalysts, achieving yields of 91–92% under optimized conditions . Industrially, DASD is a critical precursor for fluorescent whiteners, direct dyes, and corrosion inhibitors . Its disulfonic acid groups and conjugated stilbene backbone enable applications in proton exchange membranes (PEMs) and molecular electronics . Notably, DASD derivatives like 4,4′-diisothiocyanostilbene-2,2′-disulfonic acid (DIDS) are used in biochemical assays to study ion transport .
Preparation Methods
Synthetic Routes and Reaction Conditions: The synthesis of 4,4’-(1,2-Ethenediyl)bisbenzenamine typically involves the reaction of 4-nitrobenzyl chloride with ethylene diamine, followed by reduction of the resulting 4,4’-(1,2-ethanediyl)bis(4-nitrobenzene) to the corresponding diamine. The reaction conditions often require the use of solvents such as ethanol or methanol and catalysts like palladium on carbon for the reduction step.
Industrial Production Methods: In industrial settings, the production of 4,4’-(1,2-Ethenediyl)bisbenzenamine is scaled up using similar synthetic routes but optimized for higher yields and cost-effectiveness. Continuous flow reactors and automated systems are employed to ensure consistent quality and efficiency.
Chemical Reactions Analysis
Types of Reactions: 4,4’-(1,2-Ethenediyl)bisbenzenamine undergoes various chemical reactions, including:
Oxidation: The amino groups can be oxidized to nitro groups using oxidizing agents like potassium permanganate.
Reduction: The compound can be reduced to form corresponding amines using reducing agents such as lithium aluminum hydride.
Substitution: The aromatic rings can undergo electrophilic substitution reactions, such as nitration, sulfonation, and halogenation.
Common Reagents and Conditions:
Oxidation: Potassium permanganate in acidic or basic medium.
Reduction: Lithium aluminum hydride in anhydrous ether.
Substitution: Concentrated sulfuric acid for sulfonation, nitric acid for nitration, and halogens in the presence of a Lewis acid for halogenation.
Major Products Formed:
Oxidation: 4,4’-(1,2-Ethenediyl)bis(4-nitrobenzene)
Reduction: 4,4’-(1,2-Ethenediyl)bisbenzenamine
Substitution: Various substituted derivatives depending on the reagents used.
Scientific Research Applications
Chemical Properties and Structure
4,4'-Diaminostilbene is characterized by its molecular formula and consists of two amino groups attached to the para positions of the phenyl rings. The compound can also exist in various forms, including its dihydrochloride salt. Its structure facilitates interactions with other molecules, making it valuable in multiple applications.
Synthesis of Dyes and Optical Brighteners
One of the primary applications of this compound is in the synthesis of dyes and fluorescent whitening agents. The compound serves as a key intermediate in the production of various direct dyes used in textiles and paper industries.
Dye Production
- Synthesis Process : The synthesis involves bis-diazotization of this compound-2,2'-disulfonic acid, followed by coupling reactions to produce symmetrical disazo dyes. These dyes exhibit vibrant colors and are utilized extensively due to their stability and effectiveness.
- Case Study : A study demonstrated the synthesis of two new symmetrical disazo direct dyes derived from this compound-2,2'-disulfonic acid. The resulting dyes were characterized using thin-layer chromatography and spectroscopic techniques, confirming their potential for commercial applications .
Dye Name | Color | Stability | Application Area |
---|---|---|---|
Dye I | Red | High | Textiles |
Dye II | Blue | Medium | Paper |
Optical Brightening Agents
This compound is widely used as an optical brightener in laundry detergents and paper products. It absorbs ultraviolet light and re-emits it as visible blue light, enhancing the whiteness of materials.
Biological Research Applications
In addition to its industrial uses, this compound has been investigated for its biological effects.
Toxicological Studies
- Animal Studies : Toxicology studies conducted on rodents revealed that high doses of this compound can cause adverse effects such as diarrhea and emaciation . These findings underscore the importance of dosage in research applications.
Pharmacological Potential
- Drug Development : Research has explored the potential use of this compound in drug development due to its ability to interact with cellular targets. Its mechanism involves forming covalent bonds with proteins and enzymes .
Mechanism of Action
The mechanism of action of 4,4’-(1,2-Ethenediyl)bisbenzenamine involves its interaction with various molecular targets and pathways. The amino groups can form hydrogen bonds and interact with nucleophilic sites on biological molecules, influencing their structure and function. Additionally, the compound can undergo metabolic transformations, leading to the formation of active metabolites that exert specific biological effects .
Comparison with Similar Compounds
Comparison with Similar Compounds
4,4’-Diaminoazobenzene (DA)
- Structural Difference : DA replaces DASD’s ethylene (-CH=CH-) group with an azo (-N=N-) linkage.
- Functional Performance: Corrosion Inhibition: DA exhibits superior inhibition efficiency (IE) for aluminum alloys in HCl compared to DASD (DS). The electronegative -N=N- group in DA enhances adsorption via π-electrons and lone pairs on nitrogen, forming a stable protective film. EIS studies confirm DA’s higher charge-transfer resistance (Rct) and lower double-layer capacitance (Cdl) .
4,4’-Diaminodiphenyl Ether-2,2’-Disulfonic Acid (ODADS)
- Application in PEMs : ODADS and DASD are sulfonated diamines used in sulfonated polyimides (SPIs).
- Proton Conductivity : ODADS-based SPIs exhibit moderate proton conductivity (0.1–0.2 S/cm at 80°C), while DASD-derived SPIs (DSDSA) show higher conductivity due to enhanced sulfonic acid group mobility .
- Mechanical Strength : DASD’s conjugated stilbene backbone provides greater rigidity, improving mechanical stability in PEMs compared to ODADS .
4,4’-Dinitrostilbene-2,2’-Disulfonic Acid (DNS)
- Synthetic Relationship : DNS is the nitro precursor to DASD. Hydrogenation of DNS over Pd-Cu/sibunite selectively yields DASD .
- Biological Activity: DNS derivatives like Brilliant Yellow (IC₅₀ = 0.044 μM) outperform DASD derivatives (e.g., 4,4’-diaminostilbene-2,2’-disulfonate, IC₅₀ >50 μM) in inhibiting vesicular glutamate transport, highlighting the critical role of nitro groups in bioactivity .
Triazinylaminostilbenes
- Optical Brighteners: Triazinyl derivatives of DASD dominate industrial whitening agents for textiles and paper. The triazine ring enhances UV absorption and fluorescence quantum yield compared to non-derivatized DASD .
- Environmental Impact : DASD-based triazinyl whiteners are biodegradable under specific conditions, reducing ecological toxicity compared to benzidine-derived analogs .
3,3’-Diaminostilbene and Meta-Substituted Analogs
- Photophysical Properties: Singlet Lifetime: 4,4’-Diaminostilbene exhibits shorter singlet lifetimes (1–5 ns) due to higher fluorescence and intersystem crossing rates. In contrast, 3,3’-diaminostilbene has longer lifetimes (>10 ns) due to restricted torsional motion around the central double bond . Application in Electronics: 4,4’-Diaminostilbene demonstrates voltage-dependent conductance in molecular junctions, with differential conductance increasing by 40% at 450 mV.
Data Tables
Table 1: Corrosion Inhibition Efficiency of DASD vs. DA in 1 M HCl (Aluminum Alloy AA2024)
Compound | Inhibition Efficiency (%) | Rct (Ω·cm²) | Cdl (μF/cm²) |
---|---|---|---|
4,4’-Diaminostilbene (DS) | 78–82 | 1,200 | 25 |
4,4’-Diaminoazobenzene (DA) | 88–92 | 2,500 | 12 |
Table 2: IC₅₀ Values for Stilbene Derivatives in Vesicular Glutamate Uptake Inhibition
Compound | IC₅₀ (μM) |
---|---|
Brilliant Yellow | 0.044 |
4,4’-Dinitrostilbene-2,2’-disulfonate | 1.69 |
4,4’-Diaminostilbene-2,2’-disulfonate | >50 |
Key Research Findings
- Synthesis : Pd-Cu/sibunite catalysts enable scalable DASD production with minimal byproducts, addressing historical challenges in DNS hydrogenation .
- Electronics : Conjugated DASD derivatives exhibit superior charge transport in molecular junctions, making them candidates for organic semiconductors .
Q & A
Basic Research Questions
Q. What are the standard synthetic routes for preparing DSD acid and its derivatives in dye chemistry?
DSD acid is synthesized via sulfonation of 4,4'-diaminostilbene, but its derivatives (e.g., fluorescent whitening agents) often involve stepwise reactions with triazine compounds. A common method includes:
- Step 1 : React cyanuric chloride with sulfonated naphthols (e.g., H acid, I acid) to form triazinyl intermediates.
- Step 2 : Couple these intermediates with diazotized DSD acid under controlled pH (8–9) and temperature (0–5°C).
Key reagents: Cyanuric chloride, sulfonated aromatic amines, sodium nitrite for diazotization .
Validation : Confirm purity via UV-Vis spectroscopy (λmax ~340–360 nm for triazinyl derivatives) and HPLC retention times .
Q. How is DSD acid utilized in fluorescent whitening agents (FWAs), and what factors influence their efficacy?
DSD acid derivatives like 4,4′-bis(1,3,5-triazinyl)-diaminostilbene-2,2′-disulfonates are asymmetrically substituted to enhance brightness. Efficacy depends on:
- Substituent symmetry : Asymmetric triazine groups improve quantum yield by reducing aggregation.
- Application medium : Paper industry FWAs require hydrophilic modifications (e.g., polyethylene glycol grafts) for uniform fiber adhesion .
Performance metric : Compare fluorescence intensity (ex/em ~365/435 nm) against CI Fluorescent Brightener 86 .
Q. What analytical methods are used to quantify DSD acid in complex matrices?
- Spectrophotometry : Monitor absorbance at 348 nm (ε = 1.2 × 10⁴ L·mol⁻¹·cm⁻¹) in pH 7 buffer .
- HPLC : C18 column with 0.1% trifluoroacetic acid/acetonitrile gradient (retention time ~6.2 min) .
- Metal ion detection : DSD acid forms stable complexes with Cu²⁺; quantify via chelation-induced fluorescence quenching .
Advanced Research Questions
Q. How do aggregation phenomena of DSD-derived dyes affect their optical properties, and how can these be modeled?
DSD-based dyes (e.g., DSA and DAS) form H-aggregates in aqueous solutions, reducing extinction coefficients at high concentrations (>5 × 10⁻⁵ M).
- Experimental design : Vary dye concentration (10⁻⁶–10⁻³ M) and measure UV-Vis spectra. Aggregation shifts λmax and broadens peaks (e.g., DSA at 416.5 nm) .
- Matrix analysis : Use rank annihilation factor analysis (RAFA) to resolve spectral contributions from monomers, dimers, and higher aggregates. For DSA, three species dominate; DAS exhibits four .
Q. What contradictions exist in optimizing DSD acid wastewater treatment methods, and how can they be resolved?
- Fenton vs. electrochemical oxidation : Fenton’s reagent (Fe²⁺/H₂O₂) achieves 60–70% COD removal but generates sludge. Anodic oxidation (Ti/PbO₂ electrodes) removes 75–80% COD but requires high voltage (>5 V), risking electrode corrosion .
- Hybrid approach : Combine ozonation (pH 10) with granular activated carbon adsorption to achieve >90% COD reduction without secondary waste .
Q. How does plasmon coupling in DSD-functionalized nanoparticles impact their optical activity?
- Surface modification : 4,4’-diaminostilbene (without sulfonic groups) links Au/Ag nanoparticles via amine-Au bonds, inducing plasmon coupling (redshift in UV-Vis, TEM-confirmed interparticle gaps <2 nm).
- Charge transfer : Ligand-to-metal charge transfer (LMCT) occurs only in coupled regions, enhancing SERS signals (analytical enhancement factor ~10⁵) .
Q. Does thermohydrolysis of DSD acid alter its ecotoxicity profile?
Post-thermohydrolysis (300°C, supercritical water):
- Toxicity reduction : Microtox assays show LC50 increases from 12 mg/L (raw DSD acid) to >100 mg/L (hydrolyzed products).
- Biodegradability : BOD5/COD ratio improves from 0.1 to 0.4, indicating enhanced microbial degradation .
Q. What methodological challenges arise when using DSD derivatives as myelination probes in neuroimaging?
Properties
Molecular Formula |
C14H14N2 |
---|---|
Molecular Weight |
210.27 g/mol |
IUPAC Name |
4-[2-(4-aminophenyl)ethenyl]aniline |
InChI |
InChI=1S/C14H14N2/c15-13-7-3-11(4-8-13)1-2-12-5-9-14(16)10-6-12/h1-10H,15-16H2 |
InChI Key |
KOGDFDWINXIWHI-UHFFFAOYSA-N |
Canonical SMILES |
C1=CC(=CC=C1C=CC2=CC=C(C=C2)N)N |
Origin of Product |
United States |
Synthesis routes and methods
Procedure details
Disclaimer and Information on In-Vitro Research Products
Please be aware that all articles and product information presented on BenchChem are intended solely for informational purposes. The products available for purchase on BenchChem are specifically designed for in-vitro studies, which are conducted outside of living organisms. In-vitro studies, derived from the Latin term "in glass," involve experiments performed in controlled laboratory settings using cells or tissues. It is important to note that these products are not categorized as medicines or drugs, and they have not received approval from the FDA for the prevention, treatment, or cure of any medical condition, ailment, or disease. We must emphasize that any form of bodily introduction of these products into humans or animals is strictly prohibited by law. It is essential to adhere to these guidelines to ensure compliance with legal and ethical standards in research and experimentation.