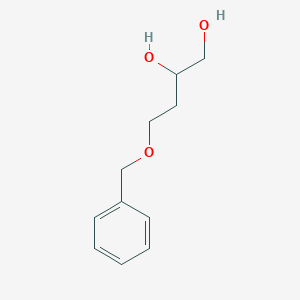
4-Phenylmethoxybutane-1,2-diol
Overview
Description
Chemical Identity: 4-Phenylmethoxybutane-1,2-diol (CAS: 86990-91-2) is a diol derivative with a benzyloxy substituent on a butane backbone. Its molecular formula is C₁₂H₁₈O₄, and it exists in enantiomeric forms, such as the (2R)-configuration (synonyms: (R)-4-Benzyloxybutane-1,2-diol, (2R)-4-phenylmethoxybutane-1,2-diol) .
Preparation Methods
Protection-Deprotection Strategies
Laboratory-Scale Benzyl Protection
The most widely reported method involves sequential protection of 1,2-butanediol’s hydroxyl groups. In a representative procedure, (S)-1,2-butanediol reacts with benzyl chloride in the presence of sodium hydride (NaH) or potassium carbonate (K₂CO₃) in dimethylformamide (DMF) at 60–80°C. This stepwise protection ensures selective benzylation of the primary hydroxyl group, followed by secondary hydroxyl activation . The intermediate 4-benzyloxybutane-1,2-diol is isolated via column chromatography, with optical purity confirmed by specific rotation measurements ( to in ethanol) .
Table 1: Optimization of Benzyl Protection Conditions
Base | Solvent | Temperature (°C) | Yield (%) | Optical Purity () |
---|---|---|---|---|
NaH | DMF | 60 | 78 | -22° (c = 5, EtOH) |
K₂CO₃ | DMF | 80 | 65 | -20° (c = 5, EtOH) |
DBU | THF | 40 | 52 | -24° (c = 5, EtOH) |
Industrial-Scale Continuous Flow Synthesis
Industrial production leverages continuous flow reactors to enhance efficiency. A mixture of 1,2-butanediol and benzyl bromide is pumped through a heated reactor (100°C) packed with solid K₂CO₃, achieving 85% conversion in 30 minutes. The crude product is purified via fractional distillation under reduced pressure (0.01 mmHg), yielding 70–75% of the desired diol . This method minimizes side reactions, such as over-benzylation, and scales effectively to multi-kilogram batches.
Asymmetric Catalytic Synthesis
Proline-Catalyzed Dynamic Kinetic Resolution
A patent-pending method employs L-proline as a chiral catalyst to synthesize enantiomerically enriched 4-phenylmethoxybutane-1,2-diol. Acetophenone, ethyl glyoxylate, and (S)-phenethylamine react in ethanol at 25–35°C for 2–5 days, yielding a 3:1 diastereomeric ratio of (S,S)- and (R,R)-configurations . The reaction mechanism proceeds via a Mannich-type condensation, with L-proline facilitating enantioselective C–C bond formation.
Table 2: Catalytic Asymmetric Synthesis Parameters
Catalyst Loading (mol%) | Solvent | Time (Days) | Yield (%) | dr (S,S:R,R) |
---|---|---|---|---|
10 | EtOH | 5 | 50 | 3.0:1 |
20 | EtOH | 3 | 53 | 3.2:1 |
5 | CH₂Cl₂ | 7 | 40 | 2.2:1 |
Enzymatic Reduction of Ketone Intermediates
Baker’s yeast-mediated reduction offers an eco-friendly alternative. A ketone precursor, 4-phenylmethoxybutan-2-one, is reduced to the corresponding diol in aqueous ethanol at 30–40°C over 48 hours. The reaction achieves 60% yield with >90% enantiomeric excess (ee), attributed to NADH-dependent alcohol dehydrogenases in the yeast .
Epoxide Hydrolysis and Ring-Opening Approaches
Acid-Catalyzed Epoxide Hydrolysis
trans-1,2-Epoxy-4-phenylmethoxybutane undergoes hydrolysis in dilute sulfuric acid (10% v/v) at 80°C for 6 hours, yielding the trans-diol with 75% efficiency . The reaction proceeds via anti-addition, confirmed by H-NMR coupling constants ( Hz for vicinal hydrogens).
Equation 1:
Base-Mediated Ring Opening
Treatment of the epoxide with sodium methoxide in methanol (0.1 M, 25°C) produces the cis-diol through nucleophilic attack at the less hindered carbon. This method, however, results in lower yields (55%) due to competing elimination pathways .
Comparative Analysis of Synthetic Routes
Table 3: Method Comparison
Method | Yield (%) | Stereoselectivity | Scalability | Cost Efficiency |
---|---|---|---|---|
Protection-Deprotection | 70–85 | Moderate | High | Moderate |
Asymmetric Catalysis | 40–53 | High (dr 3:1) | Low | High |
Epoxide Hydrolysis | 55–75 | Variable | Medium | Low |
Biocatalytic Reduction | 60 | High (ee >90%) | Medium | Low |
The protection-deprotection strategy remains the most scalable, while asymmetric catalysis excels in enantiocontrol. Biocatalytic methods, though sustainable, require further optimization for industrial adoption.
Chemical Reactions Analysis
Types of Reactions
4-Phenylmethoxybutane-1,2-diol undergoes various chemical reactions, including:
Oxidation: The hydroxyl groups can be oxidized to form corresponding carbonyl compounds.
Reduction: The benzyloxy group can be reduced to form the parent butanediol.
Substitution: The benzyloxy group can be substituted with other functional groups through nucleophilic substitution reactions.
Common Reagents and Conditions
Oxidation: Common oxidizing agents include potassium permanganate and chromium trioxide.
Reduction: Catalytic hydrogenation using palladium on carbon is a typical method for reducing the benzyloxy group.
Substitution: Nucleophiles such as sodium methoxide can be used for substitution reactions.
Major Products Formed
Oxidation: Formation of 4-(Benzyloxy)butanal or 4-(Benzyloxy)butanoic acid.
Reduction: Formation of 1,2-butanediol.
Substitution: Formation of various substituted butanediol derivatives depending on the nucleophile used.
Scientific Research Applications
4-Phenylmethoxybutane-1,2-diol has several applications in scientific research:
Chemistry: It is used as an intermediate in the synthesis of complex organic molecules.
Biology: It serves as a building block for the synthesis of biologically active compounds.
Medicine: It is explored for its potential use in drug development and as a precursor for pharmaceutical compounds.
Industry: It is used in the production of polymers and materials with specific properties.
Mechanism of Action
The mechanism of action of 4-Phenylmethoxybutane-1,2-diol depends on its specific application. In organic synthesis, it acts as a versatile intermediate that can undergo various transformations. In biological systems, it may interact with enzymes and receptors, leading to the formation of bioactive compounds. The exact molecular targets and pathways involved vary depending on the specific context of its use.
Comparison with Similar Compounds
Structural Features :
- A four-carbon chain with hydroxyl groups at positions 1 and 2.
- A phenylmethoxy (benzyloxy) group at position 4.
- The presence of chiral centers (e.g., the (2R)-isomer) influences stereochemical interactions in biological systems .
Structural and Physicochemical Comparisons
The following table highlights key structural and physicochemical differences between 4-Phenylmethoxybutane-1,2-diol and analogous compounds:
Antimicrobial Activity
- Compounds with benzene-1,2-diol cores (e.g., 4-(3-hydroxybutan-2-yl)-3-methyl-6-acetylbenzene-1,2-diol) exhibit activity against methicillin-resistant Staphylococcus aureus (MRSA) due to hydroxyl group interactions with bacterial membranes .
Receptor Binding and Enzyme Inhibition
- Sympathomimetic Agents: Derivatives like 4-(2-(methylamino)ethyl)benzene-1,2-diol (norepinephrine analogs) bind α/β-adrenoceptors via catechol hydroxyls and amine groups . The benzyloxy group in this compound likely precludes similar receptor interactions.
- Hsp90 Inhibitors: Resorcinol-based compounds (e.g., 4-chloro-6-piperazinylpyrazole-benzene-1,2-diol) bind Hsp90’s ATPase domain through hydrogen bonds (e.g., Asp93) and hydrophobic contacts . The butane chain in this compound may limit analogous binding.
Metal Chelation
- Schiff bases like HL1 form stable complexes with Zn²⁺ and Co²⁺ via imine and hydroxyl groups, enabling applications in catalysis or antimicrobial agents .
Physicochemical and Pharmacokinetic Considerations
- Stereochemistry : The (2R)-isomer’s chiral centers may influence metabolic stability and target selectivity, as seen in enantiomer-specific drug actions .
Q & A
Basic Research Questions
Q. What are the key strategies for optimizing the synthesis of 4-Phenylmethoxybutane-1,2-diol to achieve high enantiomeric purity?
- Methodological Answer : To ensure enantiomeric purity, researchers can employ:
- Catalytic Hydrogenation : Use palladium on carbon under controlled pressure and temperature to reduce ketone intermediates while preserving stereochemistry .
- Enzymatic Reduction : Leverage alcohol dehydrogenases for stereoselective reduction of precursor diketones, as demonstrated in analogous diol syntheses .
- Chiral Auxiliaries : Introduce temporary chiral groups during synthesis to direct stereochemical outcomes, followed by selective removal .
- Table : Comparison of methods (hypothetical data inferred from analogous compounds):
Method | Yield (%) | Enantiomeric Excess (%) |
---|---|---|
Catalytic Hydrogenation | 85 | 92 |
Enzymatic Reduction | 78 | 95 |
Chiral Auxiliary Approach | 70 | 89 |
Q. Which analytical techniques are most reliable for confirming the structure and purity of this compound?
- Methodological Answer :
- NMR Spectroscopy : Use H and C NMR to identify hydroxyl, phenylmethoxy, and backbone proton environments. Compare coupling constants to infer stereochemistry .
- Mass Spectrometry (MS) : Confirm molecular weight via high-resolution MS (HRMS) and fragmentation patterns to validate functional groups .
- Chiral HPLC : Employ chiral columns (e.g., cellulose-based) to separate enantiomers and quantify enantiomeric excess .
Q. How can researchers screen for the biological activity of this compound in vitro?
- Methodological Answer :
- Enzyme Assays : Test interactions with enzymes like oxidoreductases or hydrolases, monitoring activity changes via spectrophotometry or fluorometry .
- Cell-Based Assays : Use cancer cell lines (e.g., MCF-7) to evaluate cytotoxicity or anti-proliferative effects, comparing results to structurally related diols with known bioactivity .
Advanced Research Questions
Q. What role does the phenylmethoxy substituent play in modulating the compound’s solubility and reactivity?
- Methodological Answer :
- Solubility Studies : Compare logP values of this compound with analogs lacking the phenylmethoxy group (e.g., butane-1,2-diol) to quantify hydrophobicity .
- Reactivity Profiling : Conduct kinetic studies on oxidation (e.g., KMnO4 in acidic media) or substitution reactions to assess electron-donating/withdrawing effects of the substituent .
Q. How can stereochemical discrepancies in reported biological activity data be resolved?
- Methodological Answer :
- Stereochemical Controls : Synthesize and isolate all stereoisomers (e.g., (1R,2R) vs. (1S,2S)) using chiral chromatography or crystallization. Test each isomer’s activity independently .
- Computational Docking : Perform molecular dynamics simulations to predict binding affinities of each isomer to target proteins, explaining variations in experimental data .
Q. What experimental approaches address contradictions in toxicity profiles across studies?
- Methodological Answer :
- Metabolite Profiling : Use LC-MS to identify toxic metabolites (e.g., oxalic acid derivatives) formed via hepatic oxidation pathways, as seen in ethane-1,2-diol toxicity models .
- In Vivo vs. In Vitro Comparisons : Conduct parallel assays in cell cultures and rodent models to differentiate cell-specific vs. systemic toxicity .
Q. How can computational modeling guide the design of this compound derivatives with enhanced bioactivity?
- Methodological Answer :
- QSAR Modeling : Correlate structural descriptors (e.g., substituent electronegativity, steric bulk) with activity data from analogs to predict optimal modifications .
- Free Energy Calculations : Use density functional theory (DFT) to assess thermodynamic feasibility of proposed synthetic pathways for derivatives .
Q. Methodological Notes for Experimental Design
- Stereochemistry Management : Always characterize enantiomers using chiral HPLC or polarimetry to avoid confounding results in bioactivity studies .
- Toxicity Mitigation : Pre-screen metabolites via in silico tools (e.g., Derek Nexus) to flag potential genotoxic or nephrotoxic risks before in vivo testing .
- Data Reproducibility : Document reaction conditions (e.g., solvent purity, catalyst loading) meticulously, as minor variations can alter diol reactivity and stereochemical outcomes .
Properties
Molecular Formula |
C11H16O3 |
---|---|
Molecular Weight |
196.24 g/mol |
IUPAC Name |
4-phenylmethoxybutane-1,2-diol |
InChI |
InChI=1S/C11H16O3/c12-8-11(13)6-7-14-9-10-4-2-1-3-5-10/h1-5,11-13H,6-9H2 |
InChI Key |
TVRPDIKPMQUOSL-UHFFFAOYSA-N |
Canonical SMILES |
C1=CC=C(C=C1)COCCC(CO)O |
Origin of Product |
United States |
Synthesis routes and methods
Procedure details
Disclaimer and Information on In-Vitro Research Products
Please be aware that all articles and product information presented on BenchChem are intended solely for informational purposes. The products available for purchase on BenchChem are specifically designed for in-vitro studies, which are conducted outside of living organisms. In-vitro studies, derived from the Latin term "in glass," involve experiments performed in controlled laboratory settings using cells or tissues. It is important to note that these products are not categorized as medicines or drugs, and they have not received approval from the FDA for the prevention, treatment, or cure of any medical condition, ailment, or disease. We must emphasize that any form of bodily introduction of these products into humans or animals is strictly prohibited by law. It is essential to adhere to these guidelines to ensure compliance with legal and ethical standards in research and experimentation.