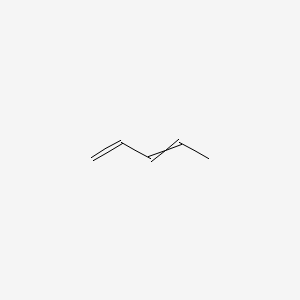
1,3-PENTADIENE
- Click on QUICK INQUIRY to receive a quote from our team of experts.
- With the quality product at a COMPETITIVE price, you can focus more on your research.
Overview
Description
1,3-PENTADIENE: C₅H₈ . It consists of two double bonds separated by a single bond, making it a conjugated system. This compound is a colorless liquid with a characteristic odor and is used as a building block in organic synthesis and polymer production .
Preparation Methods
Synthetic Routes and Reaction Conditions:
Reductive Coupling of Alkynes: One of the methods to synthesize penta-1,3-diene involves the nickel-catalyzed reductive coupling of unsymmetrical internal alkynes.
Horner–Wadsworth–Emmons Methodology: This method involves the reaction of (Z)-2-methyl-3-iodo-propenal with appropriate reagents to form the desired diene.
Industrial Production Methods: 1,3-PENTADIENE is industrially produced as a byproduct during the steam cracking of naphtha to produce ethylene. It can also be obtained from the pyrolysis of cyclopentadiene .
Chemical Reactions Analysis
Types of Reactions:
Oxidation: 1,3-PENTADIENE can undergo oxidation reactions to form various oxygenated products.
Polymerization: It can be polymerized using cationic catalytic systems based on organoaluminum compounds to form polypentadiene.
Diels-Alder Reactions: As a conjugated diene, it readily participates in Diels-Alder reactions to form cyclohexene derivatives.
Common Reagents and Conditions:
Nickel Catalysts: Used in the reductive coupling of alkynes.
Organoaluminum Compounds: Used in the cationic polymerization process.
Major Products:
Polypentadiene: Formed through polymerization.
Cyclohexene Derivatives: Formed through Diels-Alder reactions.
Scientific Research Applications
Chemistry:
Building Block: 1,3-PENTADIENE is used as a building block in the synthesis of various organic compounds.
Biology and Medicine:
Antiviral and Antibacterial Agents: Derivatives of penta-1,3-diene have shown significant antiviral and antibacterial activities
Industry:
Polymer Production: Used in the production of synthetic rubbers and other polymers.
Mechanism of Action
The mechanism of action of penta-1,3-diene and its derivatives involves interactions with specific molecular targets. For example, certain derivatives bind to the coat protein of tobacco mosaic virus, inhibiting its activity . The conjugated diene system allows for resonance stabilization, which plays a crucial role in its reactivity and interactions .
Comparison with Similar Compounds
1,3-Butadiene: Another conjugated diene with similar reactivity but a simpler structure.
2,5-Heptadiene: A nonconjugated diene with different reactivity due to the separation of double bonds by more than one single bond.
Uniqueness: 1,3-PENTADIENE’s conjugated system provides additional stability and reactivity compared to nonconjugated dienes. Its ability to participate in Diels-Alder reactions and polymerization makes it a valuable compound in both research and industrial applications .
Properties
Molecular Formula |
C5H8 |
---|---|
Molecular Weight |
68.12 g/mol |
IUPAC Name |
penta-1,3-diene |
InChI |
InChI=1S/C5H8/c1-3-5-4-2/h3-5H,1H2,2H3 |
InChI Key |
PMJHHCWVYXUKFD-UHFFFAOYSA-N |
SMILES |
CC=CC=C |
Canonical SMILES |
CC=CC=C |
Related CAS |
25212-15-1 17410-45-6 |
Origin of Product |
United States |
Retrosynthesis Analysis
AI-Powered Synthesis Planning: Our tool employs the Template_relevance Pistachio, Template_relevance Bkms_metabolic, Template_relevance Pistachio_ringbreaker, Template_relevance Reaxys, Template_relevance Reaxys_biocatalysis model, leveraging a vast database of chemical reactions to predict feasible synthetic routes.
One-Step Synthesis Focus: Specifically designed for one-step synthesis, it provides concise and direct routes for your target compounds, streamlining the synthesis process.
Accurate Predictions: Utilizing the extensive PISTACHIO, BKMS_METABOLIC, PISTACHIO_RINGBREAKER, REAXYS, REAXYS_BIOCATALYSIS database, our tool offers high-accuracy predictions, reflecting the latest in chemical research and data.
Strategy Settings
Precursor scoring | Relevance Heuristic |
---|---|
Min. plausibility | 0.01 |
Model | Template_relevance |
Template Set | Pistachio/Bkms_metabolic/Pistachio_ringbreaker/Reaxys/Reaxys_biocatalysis |
Top-N result to add to graph | 6 |
Feasible Synthetic Routes
Disclaimer and Information on In-Vitro Research Products
Please be aware that all articles and product information presented on BenchChem are intended solely for informational purposes. The products available for purchase on BenchChem are specifically designed for in-vitro studies, which are conducted outside of living organisms. In-vitro studies, derived from the Latin term "in glass," involve experiments performed in controlled laboratory settings using cells or tissues. It is important to note that these products are not categorized as medicines or drugs, and they have not received approval from the FDA for the prevention, treatment, or cure of any medical condition, ailment, or disease. We must emphasize that any form of bodily introduction of these products into humans or animals is strictly prohibited by law. It is essential to adhere to these guidelines to ensure compliance with legal and ethical standards in research and experimentation.