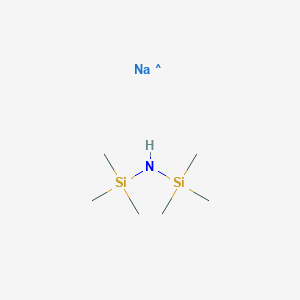
Sodium bis(trimethylsilyl)amide
- Click on QUICK INQUIRY to receive a quote from our team of experts.
- With the quality product at a COMPETITIVE price, you can focus more on your research.
Overview
Description
Preparation Methods
Synthetic Routes and Reaction Conditions: Sodium hexamethyldisilazane is typically synthesized by the reaction of hexamethyldisilazane with sodium hydride. The reaction proceeds as follows:
(CH₃)₃Si2NH+NaH→NaN(Si(CH₃)₃)₂+H₂
This reaction is usually carried out in an inert atmosphere to prevent the highly reactive sodium hydride from reacting with moisture or oxygen .
Industrial Production Methods: On an industrial scale, sodium hexamethyldisilazane is produced using similar methods but with optimized conditions to ensure high yield and purity. The use of continuous flow reactors and advanced purification techniques are common in industrial settings to achieve these goals .
Chemical Reactions Analysis
Types of Reactions: Sodium hexamethyldisilazane undergoes various types of reactions, including:
Deprotonation Reactions: It is commonly used to deprotonate weak acids, such as alcohols, amines, and ketones, to form corresponding anions.
Base-Catalyzed Reactions: It acts as a strong base in various organic reactions, including aldol condensations and Michael additions.
Common Reagents and Conditions:
Reagents: Common reagents used with sodium hexamethyldisilazane include ketones, esters, and halocarbons.
Major Products:
Enolate Derivatives: Deprotonation of ketones and esters to generate enolate derivatives.
Cyclopropanes and Cyclopropenes: Generation of carbenes by dehydrohalogenation of halocarbons, which then add to alkenes.
Scientific Research Applications
Sodium hexamethyldisilazane has a wide range of applications in scientific research:
Mechanism of Action
The primary mechanism by which sodium hexamethyldisilazane exerts its effects is through its strong basicity. It readily deprotonates weak acids, generating corresponding anions that can participate in further chemical reactions. The compound’s non-nucleophilic nature ensures that it does not interfere with the reaction substrates .
Comparison with Similar Compounds
Lithium bis(trimethylsilyl)amide (LiN(Si(CH₃)₃)₂): Similar in structure and reactivity but uses lithium instead of sodium.
Potassium bis(trimethylsilyl)amide (KN(Si(CH₃)₃)₂): Another similar compound that uses potassium.
Uniqueness: Sodium hexamethyldisilazane is unique due to its balance of strong basicity and non-nucleophilic nature, making it highly effective in deprotonation reactions without interfering with the reaction substrates .
Properties
Molecular Formula |
C6H19NNaSi2 |
---|---|
Molecular Weight |
184.38 g/mol |
InChI |
InChI=1S/C6H19NSi2.Na/c1-8(2,3)7-9(4,5)6;/h7H,1-6H3; |
InChI Key |
QKNDAUTYSODFJV-UHFFFAOYSA-N |
Canonical SMILES |
C[Si](C)(C)N[Si](C)(C)C.[Na] |
Origin of Product |
United States |
Disclaimer and Information on In-Vitro Research Products
Please be aware that all articles and product information presented on BenchChem are intended solely for informational purposes. The products available for purchase on BenchChem are specifically designed for in-vitro studies, which are conducted outside of living organisms. In-vitro studies, derived from the Latin term "in glass," involve experiments performed in controlled laboratory settings using cells or tissues. It is important to note that these products are not categorized as medicines or drugs, and they have not received approval from the FDA for the prevention, treatment, or cure of any medical condition, ailment, or disease. We must emphasize that any form of bodily introduction of these products into humans or animals is strictly prohibited by law. It is essential to adhere to these guidelines to ensure compliance with legal and ethical standards in research and experimentation.