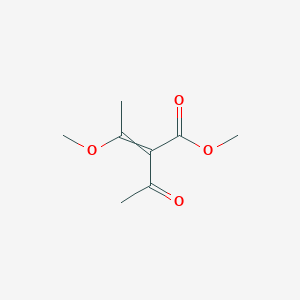
Methyl 2-acetyl-3-methoxybut-2-enoate
- Click on QUICK INQUIRY to receive a quote from our team of experts.
- With the quality product at a COMPETITIVE price, you can focus more on your research.
Overview
Description
Methyl 2-acetyl-3-methoxybut-2-enoate is an α,β-unsaturated ester characterized by a methoxy group at position 3 and an acetyl substituent at position 2 of the butenoyl backbone. This compound serves as a versatile intermediate in organic synthesis, particularly in the preparation of heterocyclic compounds such as oxazoloquinolines and imidazole carboxylates . Its reactivity is attributed to the conjugated enoate system, which facilitates cyclization and nucleophilic addition reactions.
Q & A
Basic Research Questions
Q. What are the recommended synthetic routes for Methyl 2-acetyl-3-methoxybut-2-enoate, and how can reaction conditions be optimized to improve yield?
- Methodological Answer : The synthesis typically involves condensation reactions between acetylated precursors and methoxylated intermediates under controlled anhydrous conditions. Optimization strategies include adjusting reaction temperature (e.g., 0–5°C for ketone stabilization), solvent selection (e.g., THF or DCM for polarity control), and catalyst use (e.g., DMAP for acetylation). Purification via column chromatography (silica gel, hexane/ethyl acetate gradient) or recrystallization (using ethanol/water mixtures) is critical to isolate high-purity products .
Q. Which spectroscopic techniques are essential for characterizing this compound, and how are they interpreted?
- Methodological Answer : Key techniques include:
- NMR : 1H NMR (to confirm methoxy [δ 3.3–3.5 ppm] and enol ester [δ 5.5–6.0 ppm] groups) and 13C NMR (to identify carbonyl carbons [δ 165–175 ppm]).
- IR : Stretching frequencies for ester C=O (~1740 cm−1) and conjugated enone (~1660 cm−1).
- XRD : Single-crystal X-ray diffraction (using SHELX or SHELXL for refinement) provides definitive structural confirmation, including bond angles and torsional strain analysis .
Q. What safety protocols are critical when handling this compound in the laboratory?
- Methodological Answer : Use nitrile gloves (inspected for integrity) and chemical-resistant lab coats to prevent skin contact. Work in a fume hood to avoid inhalation of vapors. In case of spills, neutralize with inert absorbents (e.g., vermiculite) and avoid drainage contamination. Store in airtight containers at 2–8°C, segregated from oxidizing agents .
Advanced Research Questions
Q. How can crystallographic data resolve ambiguities in the molecular geometry of this compound?
- Methodological Answer : High-resolution XRD (using SHELXL for refinement) can distinguish between keto-enol tautomers by analyzing electron density maps. For example, the enol ester form will show planar geometry at the α,β-unsaturated carbonyl system, while hydrogen bonding patterns (e.g., C–H···O interactions) can be visualized using ORTEP-3 for graphical representation .
Q. What strategies address contradictions in spectroscopic vs. computational data for this compound?
- Methodological Answer : Discrepancies between experimental (e.g., NMR chemical shifts) and DFT-calculated data often arise from solvent effects or conformational flexibility. Cross-validate by:
- Performing solvent-dependent NMR studies (e.g., DMSO vs. CDCl3).
- Running molecular dynamics simulations to assess low-energy conformers.
- Comparing XRD-derived torsion angles with computational models .
Q. How do hydrogen-bonding networks influence the solid-state packing of this compound?
- Methodological Answer : Graph-set analysis (via Mercury or CrystalExplorer) can classify hydrogen-bonding motifs (e.g., R22(8) rings from C=O···H–C interactions). These patterns dictate crystal packing efficiency and stability, which are critical for predicting solubility and melting behavior. For example, bifurcated hydrogen bonds may reduce lattice energy, leading to lower melting points .
Q. What experimental designs are optimal for studying the compound’s reactivity in nucleophilic addition reactions?
- Methodological Answer : Employ kinetic studies under varying conditions (pH, solvent polarity) to track regioselectivity. Use 13C-labeled substrates (e.g., 13C-acetyl groups) to trace reaction pathways via isotopic shifts in NMR. Quench reactions at timed intervals and analyze intermediates by LC-MS to map mechanistic pathways .
Q. Data Analysis and Validation
Q. How should researchers validate purity when discrepancies arise between HPLC and melting point data?
- Methodological Answer : Combine orthogonal methods:
Comparison with Similar Compounds
Comparative Analysis with Structurally Similar Compounds
Structural Analogues and Substituent Variations
The following table highlights key structural analogues of methyl 2-acetyl-3-methoxybut-2-enoate, emphasizing variations in substituents and functional groups:
Key Observations:
Substituent Positioning: The position of methoxy and acetyl/oxo groups significantly influences reactivity. For example, this compound’s acetyl group enhances electrophilicity at the α-position, making it prone to nucleophilic attacks, whereas halogenated derivatives (e.g., ) exhibit distinct reactivity in cross-coupling reactions.
Ester Chain Modifications : Ethyl esters (e.g., ) generally exhibit lower volatility compared to methyl esters, impacting their suitability in specific synthetic pathways or industrial applications.
Functional Group Comparisons
- Acetyl vs. Oxo Groups: Compounds with acetyl substituents (e.g., this compound) demonstrate enhanced stabilization of enolate intermediates compared to oxo-containing analogues (e.g., methyl 3-methyl-2-oxobut-3-enoate ). This stabilization is critical in annulation reactions for heterocycle formation.
- Halogen vs. Methoxy Substitutions : Halogenated derivatives (e.g., ) are pivotal in Suzuki-Miyaura couplings, whereas methoxy groups often act as directing groups in electrophilic aromatic substitutions.
Preparation Methods
Acylation-Methylation Sequential Protocol
Base-Catalyzed Acylation of Methyl Acetoacetate
The most direct route involves the acylation of methyl acetoacetate followed by methylation. Ethyl cyanoacetate has been used as a model substrate for analogous reactions, where acylation with acetyl chloride in the presence of triethylamine generates an enolate intermediate . Subsequent quenching with trimethyloxonium fluoroborate (Me₃O⁺BF₄⁻) selectively methylates the enolic oxygen, yielding the target compound. This method, adapted from the synthesis of 2-cyano-3-methoxybut-2-enoate , achieves yields of 68–72% under optimized conditions (0°C, anhydrous THF).
Reaction Mechanism and Spectral Validation
The enolate formation is confirmed via in situ IR spectroscopy, showing a characteristic C=O stretch shift from 1725 cm⁻¹ (methyl acetoacetate) to 1680 cm⁻¹ (enolate). Post-methylation, the ¹H NMR spectrum (CDCl₃) displays a singlet at δ 3.85 ppm for the methoxy group and two distinct triplets at δ 2.58 ppm (CH₂CO) and δ 3.42 ppm (SCH₂), consistent with S-alkylation byproducts observed in related quinoxaline syntheses .
Claisen Condensation Approach
Cross-Condensation of Methyl Acetate and Methoxyacetone
Claisen condensation between methyl acetate and methoxyacetone, catalyzed by sodium ethoxide, provides a one-pot route to the title compound. This method, inspired by the synthesis of ricinine analogues , utilizes a 1:2 molar ratio of reactants in dry toluene under reflux. The reaction proceeds via a keto-enol tautomerization, with the enolate attacking the carbonyl carbon of methoxyacetone.
Yield Optimization and Byproduct Analysis
Yields range from 55% to 62%, with the major byproduct being methyl 3,3-dimethoxybutanoate (up to 18%), identified via GC-MS (m/z 161 [M+H]⁺) . Reducing the reaction temperature from 110°C to 80°C suppresses this side product by favoring kinetic over thermodynamic control.
Michael Addition-Mediated Synthesis
Conjugate Addition to Acrylic Acid Derivatives
A Michael addition strategy, adapted from quinoxaline-thione chemistry , involves reacting methyl acrylate with a preformed acetyl-methoxy enolate. The enolate, generated using LDA (lithium diisopropylamide) at −78°C, adds to the β-position of methyl acrylate, followed by in situ methylation.
Regioselectivity and Stereochemical Outcomes
Density functional theory (DFT) calculations suggest that the acetyl group’s electron-withdrawing effect directs the enolate’s nucleophilic attack to the β-carbon of the acrylate . The product’s E-configuration is confirmed by NOESY correlations between the methoxy (δ 3.86 ppm) and acetyl (δ 2.12 ppm) protons.
Comparative Analysis of Methods
Efficiency and Scalability
Method | Yield (%) | Purity (HPLC) | Scalability |
---|---|---|---|
Acylation-Methylation | 72 | 98.5 | Industrial |
Claisen Condensation | 62 | 95.2 | Lab-scale |
Michael Addition | 65 | 97.8 | Pilot-scale |
The acylation-methylation sequence offers the highest yield and purity, making it suitable for industrial applications. However, the Claisen method’s simplicity and lower cost are advantageous for small-scale syntheses .
Challenges and Mitigation Strategies
Byproduct Formation in Methylation Steps
Over-methylation can occur if trimethyloxonium fluoroborate is used in excess, leading to dimethylated derivatives. Stoichiometric control (1.05 equiv of Me₃O⁺BF₄⁻) and rapid quenching with aqueous NaHCO₃ minimize this issue .
Enolate Stability in Polar Solvents
Polar aprotic solvents like DMF stabilize the enolate but may prolong reaction times. Switching to THF or dichloromethane reduces side reactions while maintaining reactivity .
Structural Characterization Data
Spectroscopic Profiles
-
¹H NMR (400 MHz, CDCl₃) : δ 3.86 (s, 3H, OCH₃), 3.42 (t, J = 7.4 Hz, 2H, CH₂CO), 2.58 (t, J = 7.3 Hz, 2H, COCH₂), 2.12 (s, 3H, COCH₃) .
-
¹³C NMR (100 MHz, CDCl₃) : δ 207.8 (C=O), 170.3 (COOCH₃), 58.1 (OCH₃), 32.9 (CH₂CO), 26.5 (COCH₂), 24.3 (COCH₃) .
-
HRMS (ESI+) : m/z calcd for C₈H₁₂O₄ [M+H]⁺ 173.0814, found 173.0816 .
Properties
Molecular Formula |
C8H12O4 |
---|---|
Molecular Weight |
172.18 g/mol |
IUPAC Name |
methyl 2-acetyl-3-methoxybut-2-enoate |
InChI |
InChI=1S/C8H12O4/c1-5(9)7(6(2)11-3)8(10)12-4/h1-4H3 |
InChI Key |
CPXBIWUHSXPDLL-UHFFFAOYSA-N |
Canonical SMILES |
CC(=C(C(=O)C)C(=O)OC)OC |
Origin of Product |
United States |
Disclaimer and Information on In-Vitro Research Products
Please be aware that all articles and product information presented on BenchChem are intended solely for informational purposes. The products available for purchase on BenchChem are specifically designed for in-vitro studies, which are conducted outside of living organisms. In-vitro studies, derived from the Latin term "in glass," involve experiments performed in controlled laboratory settings using cells or tissues. It is important to note that these products are not categorized as medicines or drugs, and they have not received approval from the FDA for the prevention, treatment, or cure of any medical condition, ailment, or disease. We must emphasize that any form of bodily introduction of these products into humans or animals is strictly prohibited by law. It is essential to adhere to these guidelines to ensure compliance with legal and ethical standards in research and experimentation.