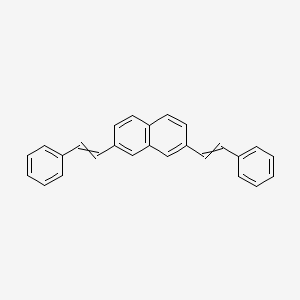
2,7-Distyrylnaphthalene
- Click on QUICK INQUIRY to receive a quote from our team of experts.
- With the quality product at a COMPETITIVE price, you can focus more on your research.
Overview
Description
2,7-Distyrylnaphthalene (DSN) is a conjugated aromatic compound characterized by a naphthalene core symmetrically substituted with two styryl groups at the 2- and 7-positions. Its structure enables strong fluorescence, making it valuable in bioimaging and materials science. The compound exhibits high photostability and tunable emission properties depending on substituents and environmental conditions (e.g., pH, solvent polarity) .
Key derivatives include:
- DSNN-NMe3+, DSNN-P, DSNN-Mor, and DSNN-Py+: These derivatives are conjugated oligoelectrolytes (COEs) with distinct functional groups (e.g., trimethylammonium, phosphonate, morpholine) that influence solubility and cellular uptake. They stain membranous structures in eukaryotic cells with minimal cytotoxicity (<20% viability loss at 1–10 µM) across diverse cell types (HeLa, HUVEC, fibroblasts) .
- Neutral DSN derivatives: Exhibit fluorescence quantum yields (Φ) up to 0.49 in non-polar solvents like xylene, with Stokes shifts ~5930 cm⁻¹ .
Preparation Methods
Synthetic Routes and Reaction Conditions
The synthesis of 2,7-Distyrylnaphthalene typically involves the Suzuki coupling reaction. This method includes the reaction between 2,7-dibromonaphthalene and a boronic acid intermediate under palladium-catalyzed conditions . The reaction is carried out in the presence of a base, such as potassium carbonate, and a solvent like tetrahydrofuran. The reaction mixture is heated to reflux, and the product is purified through column chromatography.
Industrial Production Methods
While specific industrial production methods for this compound are not extensively documented, the general approach would involve scaling up the laboratory synthesis methods. This includes optimizing reaction conditions to ensure high yield and purity, using industrial-grade reagents, and employing large-scale reactors and purification systems.
Chemical Reactions Analysis
Types of Reactions
2,7-Distyrylnaphthalene undergoes various chemical reactions, including:
Oxidation: This reaction can be carried out using oxidizing agents like potassium permanganate or chromium trioxide, leading to the formation of naphthoquinone derivatives.
Reduction: Reduction reactions can be performed using reducing agents such as lithium aluminum hydride or sodium borohydride, resulting in the formation of dihydro derivatives.
Substitution: Electrophilic substitution reactions can occur at the aromatic ring, where reagents like bromine or nitric acid can introduce substituents like bromine or nitro groups.
Common Reagents and Conditions
Oxidation: Potassium permanganate in an acidic medium.
Reduction: Lithium aluminum hydride in anhydrous ether.
Substitution: Bromine in carbon tetrachloride for bromination.
Major Products Formed
Oxidation: Naphthoquinone derivatives.
Reduction: Dihydro derivatives.
Substitution: Brominated or nitrated naphthalene derivatives.
Scientific Research Applications
2,7-Distyrylnaphthalene has several scientific research applications:
Organic Electronics: It is used in the fabrication of organic field-effect transistors (OFETs) due to its excellent charge transport properties.
Fluorescent Probes: The compound’s strong fluorescence makes it suitable for use as a fluorescent probe in biological imaging and diagnostics.
Optoelectronic Devices: It is employed in the development of organic light-emitting diodes (OLEDs) and other optoelectronic devices due to its high photoluminescence efficiency.
Mechanism of Action
The mechanism of action of 2,7-Distyrylnaphthalene in its applications is primarily based on its electronic properties. The compound exhibits strong intramolecular charge transfer (ICT) due to the presence of electron-donating and electron-accepting groups. This ICT character is crucial for its performance in optoelectronic devices, where it facilitates efficient charge transport and light emission .
Comparison with Similar Compounds
2,6-Distyrylnaphthalene Derivatives
The 2,6-isomer, particularly the donor-π-acceptor (D-π-A) derivative ASDSN, differs significantly in photophysical behavior:
Key Distinctions :
- ASDSN’s sulfonyl and amino groups enable intramolecular charge transfer (ICT), resulting in pH-dependent emission. Protonation at acidic pH induces a 132 nm blue shift, facilitating WLE .
- 2,7-DSN derivatives lack this halochromism but excel in universal cell staining due to lower cytotoxicity .
Carbazole Derivatives (2,7 vs. 3,6 Isomers)
Carbazole-based compounds are prominent in optoelectronics. Compared to 3,6-carbazole, 2,7-carbazole derivatives exhibit:
Other Distyryl Aromatics
- Distyrylbenzene (DSB) : Lacks the naphthalene core, resulting in shorter conjugation lengths and blue-shifted emission (λ~450 nm). Lower Φ (~0.3) compared to DSN derivatives .
- Tetrahydro-DSN Derivatives : Hydrogenation reduces rigidity, diminishing fluorescence intensity but improving solubility for organic electronics .
Q & A
Basic Research Questions
Q. What are the optimal synthetic routes for 2,7-Distyrylnaphthalene, and how can reaction conditions be controlled to improve yield?
Methodological Answer: The synthesis of this compound is commonly achieved via photocyclodehydrogenation of stilbene derivatives. For example, Method D (photocyclization under controlled UV irradiation) yields 65% product on a 1.74 mmol scale . Key parameters include:
- Reaction Solvent : Use anhydrous DMSO to minimize side reactions.
- Purification : Column chromatography on silica gel with gradient elution (hexane/ethyl acetate) ensures high purity.
- Characterization : Validate via melting point (262–264°C), IR (peaks at 966, 913 cm⁻¹ for trans-vinyl C–H), and ¹H-NMR (distinct olefinic protons at δ 7.79–7.83 ppm, J = 17.6 Hz) .
Q. Which spectroscopic and chromatographic techniques are critical for characterizing this compound and its derivatives?
Methodological Answer:
- IR Spectroscopy : Identifies functional groups (e.g., vinyl C–H stretching at ~960 cm⁻¹).
- ¹H-NMR : Resolves olefinic proton coupling patterns (J = 17.6 Hz for trans-configuration) and aromatic proton multiplicity .
- Mass Spectrometry (EI-MS) : Confirms molecular weight (base peak at m/z 332 for M⁺) and fragmentation pathways .
- HPLC : Monitors reaction progress using C18 columns with UV detection at 254 nm.
Advanced Research Questions
Q. How can researchers resolve discrepancies in spectroscopic data when synthesizing this compound derivatives?
Methodological Answer:
- Triplicate Runs : Ensure reproducibility by repeating synthesis and characterization under identical conditions.
- Cross-Validation : Combine XRD (for crystal structure validation) with computational simulations (DFT for predicted NMR/IR spectra) .
- Contamination Checks : Use GC-MS to detect volatile impurities and elemental analysis to verify stoichiometry.
Q. What computational methods are effective for modeling the charge-transfer properties of this compound in optoelectronic applications?
Methodological Answer:
- Density Functional Theory (DFT) : Calculate HOMO/LUMO energies (e.g., B3LYP/6-31G* basis set) to predict bandgap and exciton dissociation efficiency .
- Time-Dependent DFT (TD-DFT) : Simulate UV-Vis absorption spectra and compare with experimental data to validate electronic transitions.
- Molecular Dynamics : Model intermolecular stacking in bulk-heterojunction solar cells to optimize charge-carrier mobility .
Q. How should conflicting toxicity data for this compound be analyzed in environmental risk assessments?
Methodological Answer:
- Risk of Bias Assessment : Apply criteria from Table C-6/C-7 (e.g., randomization, outcome reporting) to grade study reliability .
- Confidence Rating : Categorize studies as High/Moderate/Low based on methodological rigor (e.g., High: 4/4 "Yes" in bias criteria) .
- Data Gap Prioritization : Focus on endpoints with insufficient evidence (e.g., renal effects in long-term exposure) using frameworks from Chapter 6 of ATSDR profiles .
Properties
Molecular Formula |
C26H20 |
---|---|
Molecular Weight |
332.4 g/mol |
IUPAC Name |
2,7-bis(2-phenylethenyl)naphthalene |
InChI |
InChI=1S/C26H20/c1-3-7-21(8-4-1)11-13-23-15-17-25-18-16-24(20-26(25)19-23)14-12-22-9-5-2-6-10-22/h1-20H |
InChI Key |
BAXGJINOQBOMFG-UHFFFAOYSA-N |
Canonical SMILES |
C1=CC=C(C=C1)C=CC2=CC3=C(C=C2)C=CC(=C3)C=CC4=CC=CC=C4 |
Origin of Product |
United States |
Disclaimer and Information on In-Vitro Research Products
Please be aware that all articles and product information presented on BenchChem are intended solely for informational purposes. The products available for purchase on BenchChem are specifically designed for in-vitro studies, which are conducted outside of living organisms. In-vitro studies, derived from the Latin term "in glass," involve experiments performed in controlled laboratory settings using cells or tissues. It is important to note that these products are not categorized as medicines or drugs, and they have not received approval from the FDA for the prevention, treatment, or cure of any medical condition, ailment, or disease. We must emphasize that any form of bodily introduction of these products into humans or animals is strictly prohibited by law. It is essential to adhere to these guidelines to ensure compliance with legal and ethical standards in research and experimentation.