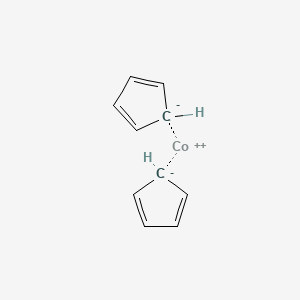
bis(cyclopenta-2,4-dien-1-yl)cobalt
Overview
Description
bis(cyclopenta-2,4-dien-1-yl)cobalt, also known as cobaltocene, is an organometallic compound with the formula Co(C₅H₅)₂. It is a member of the metallocene family, which includes compounds like ferrocene and nickelocene. This compound is known for its sandwich structure, where a cobalt atom is sandwiched between two cyclopentadienyl rings. This compound is widely used in various fields due to its unique chemical properties .
Preparation Methods
Synthetic Routes and Reaction Conditions
bis(cyclopenta-2,4-dien-1-yl)cobalt can be synthesized through the reaction of cyclopentadienyl sodium with cobalt(II) chloride in tetrahydrofuran (THF). The reaction proceeds as follows:
2C5H5Na+CoCl2→Co(C5H5)2+2NaCl
The reaction is typically carried out under an inert atmosphere to prevent oxidation .
Industrial Production Methods
Industrial production of bis(cyclopentadienyl)cobalt involves similar synthetic routes but on a larger scale. The reaction conditions are optimized to ensure high yield and purity of the product. The compound is usually purified by sublimation or recrystallization .
Chemical Reactions Analysis
Types of Reactions
bis(cyclopenta-2,4-dien-1-yl)cobalt undergoes various types of chemical reactions, including:
Oxidation: It can be oxidized to form bis(cyclopentadienyl)cobalt(III) compounds.
Reduction: It acts as a one-electron reducing agent in various reactions.
Substitution: It can undergo substitution reactions with other ligands.
Common Reagents and Conditions
Oxidation: Common oxidizing agents include oxygen and halogens.
Reduction: It is often used in the presence of reducing agents like lithium aluminum hydride.
Substitution: Ligands such as carbon monoxide can replace one or both cyclopentadienyl rings.
Major Products
Oxidation: this compound(III) compounds.
Reduction: Cobalt(I) derivatives.
Substitution: Cyclopentadienylcobalt dicarbonyl.
Scientific Research Applications
bis(cyclopenta-2,4-dien-1-yl)cobalt has numerous applications in scientific research:
Biology: It serves as a model compound for studying the behavior of metallocenes in biological systems.
Medicine: Research is ongoing to explore its potential use in medical applications, such as drug delivery systems.
Industry: It is used in the production of polymers and as a redox-active species in batteries
Mechanism of Action
bis(cyclopenta-2,4-dien-1-yl)cobalt exerts its effects through its ability to donate or accept electrons. The cobalt atom in the center of the molecule can undergo oxidation or reduction, making it a versatile redox agent. This property is utilized in various catalytic processes and redox reactions. The cyclopentadienyl rings stabilize the cobalt center, allowing it to participate in a wide range of chemical reactions .
Comparison with Similar Compounds
bis(cyclopenta-2,4-dien-1-yl)cobalt is unique among metallocenes due to its specific redox properties. Similar compounds include:
Ferrocene (Fe(C₅H₅)₂): Known for its stability and use in organic synthesis.
Nickelocene (Ni(C₅H₅)₂): Used in catalysis and as a precursor for nickel-based materials.
Bis(pentamethylcyclopentadienyl)cobalt (Co(C₅Me₅)₂): A more electron-rich analogue of bis(cyclopentadienyl)cobalt, used in electronic applications
This compound stands out due to its balance of stability and reactivity, making it a valuable compound in both research and industrial applications.
Properties
Molecular Formula |
C10H10Co |
---|---|
Molecular Weight |
189.12 g/mol |
IUPAC Name |
cobalt(2+);cyclopenta-1,3-diene |
InChI |
InChI=1S/2C5H5.Co/c2*1-2-4-5-3-1;/h2*1-5H;/q2*-1;+2 |
InChI Key |
ILZSSCVGGYJLOG-UHFFFAOYSA-N |
SMILES |
[CH-]1C=CC=C1.[CH-]1C=CC=C1.[Co+2] |
Canonical SMILES |
[CH-]1C=CC=C1.[CH-]1C=CC=C1.[Co+2] |
melting_point |
343 to 345 °F (NTP, 1992) |
physical_description |
Cobaltocene appears as black-purple crystals or black solid. Sublimes at 104 °F and 0.1 mmHg. (NTP, 1992) Black-purple or black solid; Insoluble in water; [CAMEO] Black-violet crystals; [Acros Organics MSDS] |
solubility |
less than 1 mg/mL at 70.7 °F (NTP, 1992) |
Origin of Product |
United States |
Synthesis routes and methods
Procedure details
Retrosynthesis Analysis
AI-Powered Synthesis Planning: Our tool employs the Template_relevance Pistachio, Template_relevance Bkms_metabolic, Template_relevance Pistachio_ringbreaker, Template_relevance Reaxys, Template_relevance Reaxys_biocatalysis model, leveraging a vast database of chemical reactions to predict feasible synthetic routes.
One-Step Synthesis Focus: Specifically designed for one-step synthesis, it provides concise and direct routes for your target compounds, streamlining the synthesis process.
Accurate Predictions: Utilizing the extensive PISTACHIO, BKMS_METABOLIC, PISTACHIO_RINGBREAKER, REAXYS, REAXYS_BIOCATALYSIS database, our tool offers high-accuracy predictions, reflecting the latest in chemical research and data.
Strategy Settings
Precursor scoring | Relevance Heuristic |
---|---|
Min. plausibility | 0.01 |
Model | Template_relevance |
Template Set | Pistachio/Bkms_metabolic/Pistachio_ringbreaker/Reaxys/Reaxys_biocatalysis |
Top-N result to add to graph | 6 |
Feasible Synthetic Routes
Disclaimer and Information on In-Vitro Research Products
Please be aware that all articles and product information presented on BenchChem are intended solely for informational purposes. The products available for purchase on BenchChem are specifically designed for in-vitro studies, which are conducted outside of living organisms. In-vitro studies, derived from the Latin term "in glass," involve experiments performed in controlled laboratory settings using cells or tissues. It is important to note that these products are not categorized as medicines or drugs, and they have not received approval from the FDA for the prevention, treatment, or cure of any medical condition, ailment, or disease. We must emphasize that any form of bodily introduction of these products into humans or animals is strictly prohibited by law. It is essential to adhere to these guidelines to ensure compliance with legal and ethical standards in research and experimentation.