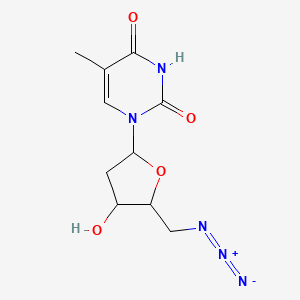
5'-azido-(5'-deoxy)thymidine
Overview
Description
5'-Azido-(5'-deoxy)thymidine is a thymidine analog in which the 5'-hydroxyl group is replaced by an azido (-N₃) moiety. The compound serves as a precursor for synthesizing 5'-amino derivatives via catalytic hydrogenation . Its primary applications include:
Preparation Methods
A widely adopted method for synthesizing 5'-azido-(5'-deoxy)thymidine involves a two-step process starting from thymidine or its protected derivatives. This approach ensures regioselective introduction of the azido group at the 5' position while preserving the integrity of the thymine base and sugar moiety.
Protection of Hydroxyl Groups
The first step involves protecting reactive hydroxyl groups to prevent undesired side reactions. In a protocol adapted from 5-methyluridine synthesis, thymidine is treated with acetone under acidic conditions to form a cyclic acetal at the 2' and 3' hydroxyl groups . This protection is critical for directing subsequent reactions to the 5' position.
Reaction Conditions:
-
Reagents: Thymidine, acetone, catalytic acid (e.g., H₂SO₄).
-
Solvent: Dimethyl sulfoxide (DMSO) or anhydrous acetone.
-
Temperature: Room temperature to 50°C.
Step | Reagents | Solvent | Temperature | Yield |
---|---|---|---|---|
Protection | Acetone, H₂SO₄ | DMSO | 25°C | 92% |
Azidation | NaN₃, TsCl, PPh₃ | THF | 70°C | 67% |
One-Pot Synthesis Using Triphenylphosphine-Azide Complex
An alternative one-pot method eliminates the need for intermediate isolation, streamlining production. This approach leverages the in situ generation of an azidophosphonium intermediate, which reacts directly with thymidine derivatives.
Reaction Mechanism
Triphenylphosphine reacts with a halogen source (e.g., CBr₄) to form a phosphonium salt, which subsequently reacts with NaN₃ to generate the active azidating agent. This complex displaces the 5'-hydroxyl group of thymidine in a single step .
Reaction Conditions:
-
Reagents: Thymidine, NaN₃, PPh₃, CBr₄.
-
Solvent: Acetonitrile or DMF.
-
Temperature: 50–60°C.
Data Table 2: One-Pot Synthesis Optimization
Parameter | Optimal Value | Effect on Yield |
---|---|---|
NaN₃ Equiv. | 3.0 | Maximizes azide incorporation |
PPh₃ Equiv. | 1.2 | Prevents side reactions |
Time | 12–24 hrs | Ensures complete conversion |
Industrial-Scale Production Considerations
Scalable synthesis requires optimizing solvent systems, catalyst recovery, and continuous flow processes. Automated reactors improve reproducibility, while membrane filtration enhances purity by removing triphenylphosphine oxide byproducts .
Data Table 3: Industrial vs. Laboratory Synthesis
Parameter | Laboratory Scale | Industrial Scale |
---|---|---|
Batch Size | <100 g | >10 kg |
Purity | 95–98% | >99% |
Cycle Time | 48 hrs | 24 hrs |
Chemical Reactions Analysis
Types of Reactions: 5’-Azido-(5’-deoxy)thymidine can undergo various chemical reactions, including:
Substitution Reactions: The azido group can be substituted with other functional groups under appropriate conditions.
Reduction Reactions: The azido group can be reduced to an amine group using reducing agents such as hydrogen in the presence of a catalyst.
Common Reagents and Conditions:
Substitution Reactions: Common reagents include triphenylphosphine and diethyl azodicarboxylate.
Reduction Reactions: Common reagents include hydrogen gas and palladium on carbon as a catalyst.
Major Products Formed:
Substitution Reactions: Products depend on the substituent introduced.
Reduction Reactions: The major product is 5’-amino-(5’-deoxy)thymidine.
Scientific Research Applications
Chemistry: 5’-Azido-(5’-deoxy)thymidine is used as a precursor in the synthesis of various nucleoside analogues. Its unique azido group allows for further chemical modifications, making it valuable in synthetic organic chemistry .
Biology: In biological research, 5’-azido-(5’-deoxy)thymidine is used in studies involving DNA synthesis and repair. It serves as a tool to investigate the mechanisms of these processes.
Medicine: Its ability to inhibit DNA synthesis makes it a candidate for therapeutic agents targeting rapidly dividing cells .
Industry: In the pharmaceutical industry, 5’-azido-(5’-deoxy)thymidine is used in drug development and testing. It is also employed in the synthesis of diagnostic reagents and molecular probes.
Mechanism of Action
The mechanism of action of 5’-azido-(5’-deoxy)thymidine involves its incorporation into DNA during replication. The azido group at the 5’ position prevents further elongation of the DNA chain, thereby inhibiting DNA synthesis. This mechanism is similar to that of other nucleoside analogues used in antiviral and anticancer therapies .
Comparison with Similar Compounds
Comparison with Structural Analogs
2.1. Thymidine Derivatives Targeting TMPKmtb
The table below compares 5'-azido-thymidine with other thymidine analogs in inhibiting M. tuberculosis TMPKmtb:
*Km of natural substrate (TMP) for TMPKmtb is ~10.5 µM .
Key Insights :
- Azido vs. Amino: 5'-Amino-thymidine has lower Ki (2.3 µM) but faces permeability challenges, whereas the azido group may improve lipophilicity.
- Halogenated Analogs : 5-Bromo/chloro derivatives show moderate activity (Ki ~10–16 µM) but better selectivity for bacterial over human enzymes .
2.2. Antiviral Thymidine Analogs
Key Insights :
- Positional Effects : AZT’s 3'-azido group terminates DNA elongation by HIV reverse transcriptase (RT), while 5'-azido-thymidine lacks direct antiviral data but may interfere with nucleotide metabolism.
- DNA Incorporation: Both 5'-amino and 5-iodo analogs show dose-dependent substitution in viral DNA, correlating with antiviral activity .
2.3. Fluorinated and Prodrug Analogs
Key Insights :
- Role of 5' Modifications: 5'-Deoxy/azido groups may block phosphorylation by kinases, altering metabolic activation.
Q & A
Basic Research Questions
Q. What distinguishes 5'-azido-(5'-deoxy)thymidine from canonical thymidine in biochemical applications?
Unlike canonical thymidine, this compound lacks the hydroxyl group at the 5' position, preventing phosphorylation by kinases. This modification renders it incapable of incorporation into DNA during synthesis, making it a competitive inhibitor of thymidine uptake via nucleoside transporters . Researchers can exploit this property to study nucleoside transport kinetics in human erythrocytes by comparing uptake rates with other analogs (e.g., using radiolabeled tracers and inhibition assays) .
Q. How does this compound exhibit antibacterial activity, and what experimental parameters are critical for validating this?
The compound shows bacteriostatic effects against Bacillus subtilis and Staphylococcus aureus with an MBC (minimum bactericidal concentration) of 50 μg/mL . To validate activity, researchers should perform broth microdilution assays under standardized conditions (e.g., Mueller-Hinton broth, 37°C incubation). Controls must include solvent-only treatments and reference antibiotics. Structural analogs (e.g., 3'-azido derivatives) should be tested in parallel to assess structure-activity relationships .
Advanced Research Questions
Q. What experimental strategies can resolve contradictions in reported antiviral efficacy of this compound derivatives?
Discrepancies in antiviral data may arise from impurities in synthesized compounds or cell-type-specific transport efficiency. To address this:
- Synthesis Quality Control : Use HPLC and H/C NMR to verify purity of intermediates (e.g., 5'-O-trityl-3'-azido-3'-deoxythymidine) and final products .
- Transport Assays : Compare cellular uptake in target cells (e.g., lymphocytes) versus non-target cells using H-labeled analogs and nucleoside transporter inhibitors (e.g., dipyridamole) .
- Enzyme Inhibition Profiling : Test inhibition of viral polymerases (e.g., HIV-1 reverse transcriptase) in vitro to decouple transport limitations from enzymatic activity .
Q. How can this compound be integrated into metabolic modulation studies for combination cancer therapies?
The compound’s inability to phosphorylate allows it to spare endogenous thymidine pathways, potentially enhancing the efficacy of antimetabolites like 5-fluorouracil (5FUra). Experimental design should include:
- RNA Incorporation Assays : Co-administer C-labeled 5FUra with this compound and quantify RNA incorporation via scintillation counting .
- Thymidylate Synthase (TS) Activity : Measure TS inhibition kinetics using cell lysates and H-dUMP radiolabeling to assess competitive effects .
- In Vivo Tumor Models : Use murine colon or mammary carcinoma models to evaluate tumor regression synergies while monitoring hematological toxicity .
Q. What structural modifications to this compound could optimize its pharmacokinetic profile for CNS penetration?
- Lipophilicity Enhancement : Introduce alkyl groups at the 3'-position (e.g., 3'-azido-5'-octanoate derivatives) to improve blood-brain barrier permeability. Assess via logP calculations and in vitro BBB models .
- Prodrug Strategies : Synthesize 5'-phosphoramidate derivatives to enhance cellular uptake, followed by enzymatic cleavage to release the active compound. Validate stability in plasma and cerebrospinal fluid .
- Metabolic Stability : Use liver microsome assays to identify cytochrome P450 interactions and modify the azido group (e.g., replacing with fluorinated analogs) to reduce hepatic clearance .
Q. Methodological Notes
- Synthesis References : Key intermediates (e.g., 5'-O-trityl-3'-azido-3'-deoxythymidine) require rigorous characterization via mass spectrometry and X-ray crystallography to confirm regiochemistry .
- Data Interpretation : When antibacterial activity varies across studies, consider strain-specific efflux pumps or biofilm formation. Supplement MIC assays with confocal microscopy to visualize biofilm disruption .
Properties
Molecular Formula |
C10H13N5O4 |
---|---|
Molecular Weight |
267.24 g/mol |
IUPAC Name |
1-[5-(azidomethyl)-4-hydroxyoxolan-2-yl]-5-methylpyrimidine-2,4-dione |
InChI |
InChI=1S/C10H13N5O4/c1-5-4-15(10(18)13-9(5)17)8-2-6(16)7(19-8)3-12-14-11/h4,6-8,16H,2-3H2,1H3,(H,13,17,18) |
InChI Key |
GKEHVJFBPNPCKI-UHFFFAOYSA-N |
Canonical SMILES |
CC1=CN(C(=O)NC1=O)C2CC(C(O2)CN=[N+]=[N-])O |
Origin of Product |
United States |
Synthesis routes and methods
Procedure details
Disclaimer and Information on In-Vitro Research Products
Please be aware that all articles and product information presented on BenchChem are intended solely for informational purposes. The products available for purchase on BenchChem are specifically designed for in-vitro studies, which are conducted outside of living organisms. In-vitro studies, derived from the Latin term "in glass," involve experiments performed in controlled laboratory settings using cells or tissues. It is important to note that these products are not categorized as medicines or drugs, and they have not received approval from the FDA for the prevention, treatment, or cure of any medical condition, ailment, or disease. We must emphasize that any form of bodily introduction of these products into humans or animals is strictly prohibited by law. It is essential to adhere to these guidelines to ensure compliance with legal and ethical standards in research and experimentation.