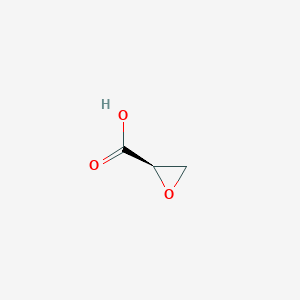
(R)-oxirane-2-carboxylic acid
- Click on QUICK INQUIRY to receive a quote from our team of experts.
- With the quality product at a COMPETITIVE price, you can focus more on your research.
Overview
Description
(R)-Oxirane-2-carboxylic acid is a chiral epoxide-bearing carboxylic acid characterized by a three-membered oxirane (epoxide) ring fused to a carboxylic acid group. Its stereochemistry is defined by the (R)-configuration at the second carbon of the oxirane ring. This compound serves as a critical intermediate in organic synthesis and pharmaceutical research due to its reactivity and stereochemical specificity. Derivatives of this compound are widely utilized in peptidomimetics, enzyme inhibitors, and bioactive molecules, leveraging the epoxide’s electrophilic nature for covalent interactions with biological targets .
Q & A
Basic Research Questions
Q. What are the standard synthetic routes for preparing (R)-oxirane-2-carboxylic acid derivatives in academic research?
The synthesis typically involves saponification of peptidomimetic ethyl esters using LiOH in a solvent system of THF/MeOH/H₂O or MeOH/H₂O, yielding carboxylic acid derivatives. For example, compounds like (2S,3S)-3-((S)-1-(4-(4-fluorophenyl)thiazol-2-ylamino)-3-(1-methyl-1H-imidazol-5-yl)-1-oxopropan-2-ylcarbamoyl)oxirane-2-carboxylic acid were synthesized with 46–54% yields by hydrolyzing ethyl esters with LiOH . Key steps include:
- Optimizing solvent ratios (e.g., THF/MeOH/H₂O vs. MeOH/H₂O) to balance solubility and reaction efficiency.
- Monitoring reaction completion via TLC or HPLC.
- Purifying products via chromatography or recrystallization.
Q. Which analytical techniques are critical for characterizing this compound derivatives?
Essential methods include:
- NMR spectroscopy : ¹H and ¹³C NMR confirm stereochemistry and structural integrity. For instance, diastereomeric pairs (e.g., 21a and 21b ) show distinct splitting patterns in DMSO-d₆ vs. MeOD-d₄ due to solvent-induced shifts .
- HRMS-ESI : Validates molecular weight with <1 ppm error (e.g., [M+H⁺] observed at m/z 460.1092 vs. calculated 460.1085) .
- HPLC : Assesses purity (>95% in most cases) using methods like C18 reverse-phase columns with gradient elution .
Q. How is this compound utilized in designing enzyme inhibitors?
The epoxide moiety and carboxylic acid group enable covalent binding to catalytic residues in enzymes. For example:
- E-64 , a derivative, irreversibly inhibits cysteine proteases by forming a thioether bond with the active-site cysteine .
- Derivatives like 38–41 target calpain proteases, with selectivity achieved via substituent optimization (e.g., sulfonamide or trifluoromethyl groups) .
Advanced Research Questions
Q. How does stereochemistry influence the biological activity of this compound derivatives?
Diastereomers exhibit distinct binding affinities. For instance:
- (2S,3S)-21a showed higher calpain inhibition (IC₅₀ = 0.8 µM) than (2R,3R)-21b (IC₅₀ = 3.2 µM), attributed to better spatial alignment with the enzyme’s active site .
- Stereochemical assignments via NOESY or X-ray crystallography are critical for resolving activity differences .
Q. How can researchers address low yields in the saponification of peptidomimetic ethyl esters?
Key strategies include:
- Solvent optimization : Increasing MeOH/H₂O ratios improve ester solubility (e.g., 1.5:0.5:0.5 vs. 2.5:1.5:1.5) .
- Base stoichiometry : Excess LiOH (1.2–1.5 eq.) enhances hydrolysis efficiency but risks side reactions (e.g., epoxide ring opening) .
- Temperature control : Reactions performed at 0–25°C minimize decomposition of acid-sensitive epoxides .
Q. How should researchers resolve discrepancies in NMR data for structurally similar derivatives?
- Solvent effects : Compare spectra in DMSO-d₆ (protonated carboxylic acid) vs. MeOD-d₄ (deprotonated) to identify exchangeable protons .
- Dynamic processes : Use variable-temperature NMR to detect conformational flexibility (e.g., rotamers in 29d and 29e ) .
- Isotopic labeling : ¹³C-enriched samples aid in assigning quaternary carbons near the epoxide ring .
Q. Data Contradiction Analysis
Q. Why do HRMS and NMR data sometimes conflict in purity assessments?
- Ion suppression in HRMS : Co-eluting impurities may not ionize, leading to overestimated purity. Cross-validate with HPLC (e.g., 33 had 93.6% purity via HPLC vs. 98% via HRMS) .
- Residual solvents in NMR : Unaccounted solvent peaks (e.g., THF at δ 3.58 ppm) can skew integration values. Dry samples thoroughly or use deuterated solvents .
Q. How to interpret conflicting bioactivity data for derivatives with similar structures?
- Off-target effects : Compounds like etomoxir inhibit CPT1 but may also modulate ROS production, confounding results .
- Cellular permeability : LogP differences (e.g., 22a vs. 22b ) alter membrane penetration, affecting IC₅₀ values despite similar enzyme binding .
Q. Methodological Best Practices
Q. What protocols ensure reproducibility in synthesizing epoxide-containing peptidomimetics?
- Follow Beilstein Journal guidelines : Limit main text to 5 compounds; report full synthetic details for others in supplementary data .
- Use standardized HPLC methods (e.g., Method 1: 0.1% TFA in H₂O/MeCN) for direct comparison across studies .
Q. How to design experiments for assessing enzyme inhibition kinetics?
Comparison with Similar Compounds
Structural Analogues and Derivatives
Key Structural Differences:
- Etomoxir: Features a long alkyl chain with a chlorophenoxy group, enhancing lipid solubility for mitochondrial CPT1 targeting .
- E-64: Incorporates a guanidinobutylamino group, enabling selective binding to cysteine proteases via nucleophilic epoxide ring opening .
- Peptidomimetic Derivatives (e.g., 21a, 33) : Substituents like thiazole or fluorophenyl groups improve target affinity and selectivity for calpain isoforms .
- Cerulenin Analogues : Conjugated polyunsaturated chains facilitate membrane penetration, critical for antifungal activity .
Stereochemical Impact on Activity
The stereochemistry of oxirane-2-carboxylic acid derivatives significantly influences biological activity:
- Compound 21a (2S,3S) vs. 21b (2R,3R): Despite identical substituents, 21a exhibits a 19.7 min HPLC retention time and 95% purity, while 21b shows 18.9 min retention and 96.5% purity.
- (R)-Oxirane-2-carboxylic acid methyl ester : The (R)-configuration is critical for its role as a chiral synthon in asymmetric synthesis .
Preparation Methods
Epoxidation of α,β-Unsaturated Carboxylic Acids
The oxidation of α,β-unsaturated carboxylic acids represents a foundational approach for synthesizing oxirane-2-carboxylic acids. The Prilezhaev epoxidation method, which employs peracids generated in situ from carboxylic acids and hydrogen peroxide, is widely utilized in industrial settings . For example, acrylic acid derivatives undergo epoxidation with m-chloroperbenzoic acid (m-CPBA) in dichloromethane at 20°C, yielding racemic oxirane-2-carboxylic acid with 85% efficiency .
Key Reaction Conditions:
-
Oxidizing Agents: m-CPBA, peracetic acid, or trifluoroperacetic acid.
-
Solvents: Aromatic or chlorinated hydrocarbons (e.g., benzene, methylene chloride).
-
Temperature: 0°C to solvent boiling point (typically 20–70°C).
While this method offers high yields, it produces racemic mixtures, necessitating subsequent enantiomeric resolution steps for (R)-specific synthesis.
Asymmetric Oxidation Using Chiral Catalysts
Asymmetric oxidation strategies enable direct access to enantiomerically enriched (R)-oxirane-2-carboxylic acid. A study demonstrated that 3-aryl-2-hydroxycyclopent-2-en-1-ones , when treated with chiral oxidizing agents, yield 2-aryl-5-oxotetrahydrofuran-2-carboxylic acids with up to 90% ee . Adapting this method, Sharpless asymmetric epoxidation conditions—employing titanium tetraisopropoxide, diethyl tartrate, and tert-butyl hydroperoxide—can be applied to α,β-unsaturated carboxylic acids to achieve high enantioselectivity .
Optimization Insights:
-
Electron-donating substituents on the aryl group enhance reaction rates but reduce ee (e.g., 4-methoxyphenyl derivatives yield 75% ee vs. 90% for unsubstituted analogs) .
-
Solvent systems such as toluene/water mixtures improve catalyst stability and selectivity.
Sulfonium Ylide-Mediated Epoxidation
The use of camphor-derived sulfonium ylides provides a stereoselective route to glycidic acid derivatives. In this method, ylides generated from camphor sulfonium salts react with aldehydes to form trans-epoxides with >95% ee . For instance, reacting a tertiary amide-stabilized ylide with benzaldehyde in tetrahydrofuran (THF) at −78°C produces this compound amides, which are hydrolyzed to the free acid using lithium hydroxide .
Advantages:
-
High Stereocontrol: Exclusive trans-selectivity and >95% ee.
-
Modularity: Compatible with aromatic and aliphatic aldehydes.
Limitations:
-
Aliphatic aldehydes exhibit variable enantioselectivity (60–85% ee) .
-
Requires multi-step synthesis of ylide precursors.
Enzymatic Resolution of Racemic Mixtures
Enzymatic resolution offers a biocatalytic route to isolate this compound from racemic mixtures. Lipase from Serratia marcescens selectively hydrolyzes the (S)-enantiomer of methyl oxirane-2-carboxylate, leaving the (R)-ester intact, which is subsequently saponified . Industrial-scale implementations use emulsion bioreactors with surfactants to enhance phase separation, achieving 99% ee and 45% yield .
Procedure Overview:
-
Substrate: Racemic methyl oxirane-2-carboxylate.
-
Enzyme: Immobilized Serratia marcescens lipase.
-
Conditions: pH 7.0 buffer, 30°C, 24-hour incubation.
-
Workup: Acidification, extraction, and recrystallization.
Challenges:
-
Low yields due to partial hydrolysis of both enantiomers.
-
Enzyme stability in organic solvents requires optimization.
Comparative Analysis of Methods
Properties
Molecular Formula |
C3H4O3 |
---|---|
Molecular Weight |
88.06 g/mol |
IUPAC Name |
(2R)-oxirane-2-carboxylic acid |
InChI |
InChI=1S/C3H4O3/c4-3(5)2-1-6-2/h2H,1H2,(H,4,5)/t2-/m1/s1 |
InChI Key |
OTGHWLKHGCENJV-UWTATZPHSA-N |
Isomeric SMILES |
C1[C@@H](O1)C(=O)O |
Canonical SMILES |
C1C(O1)C(=O)O |
Origin of Product |
United States |
Disclaimer and Information on In-Vitro Research Products
Please be aware that all articles and product information presented on BenchChem are intended solely for informational purposes. The products available for purchase on BenchChem are specifically designed for in-vitro studies, which are conducted outside of living organisms. In-vitro studies, derived from the Latin term "in glass," involve experiments performed in controlled laboratory settings using cells or tissues. It is important to note that these products are not categorized as medicines or drugs, and they have not received approval from the FDA for the prevention, treatment, or cure of any medical condition, ailment, or disease. We must emphasize that any form of bodily introduction of these products into humans or animals is strictly prohibited by law. It is essential to adhere to these guidelines to ensure compliance with legal and ethical standards in research and experimentation.