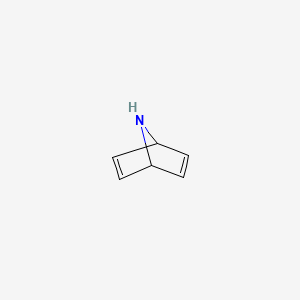
7-Azanorbornadiene
- Click on QUICK INQUIRY to receive a quote from our team of experts.
- With the quality product at a COMPETITIVE price, you can focus more on your research.
Overview
Description
7-Azanorbornadiene (7-ANBD) is a bicyclic compound with the molecular formula C₆H₇N, featuring a nitrogen atom at the bridgehead position of a norbornadiene framework. Its strained bicyclic structure and electron-deficient alkene make it highly reactive in cycloaddition and bioorthogonal chemistry . The compound was first synthesized via Diels-Alder reactions involving fulvenes and benzyne intermediates, as described by Warrener et al. . Key structural features include:
- Strained Alkene: The [2.2.1] bicyclic system imposes significant ring strain (~20 kcal/mol), enhancing its reactivity in inverse electron-demand Diels-Alder (iEDDA) reactions .
- Nitrogen Lone Pair Orientation: Photoelectron spectroscopy (PE) reveals that the nitrogen lone pair interacts with adjacent π bonds, with syn-isomers exhibiting stronger through-space interactions (0.98 eV splitting between PE bands) compared to anti-isomers .
Scientific Research Applications
Organic Synthesis
7-Azanorbornadiene serves as a valuable intermediate in organic synthesis due to its ability to undergo various transformations:
- Diels-Alder Reactions : This compound can act as a diene in Diels-Alder reactions, forming complex cyclic structures that are useful in the synthesis of natural products and pharmaceuticals.
- Nitrene Chemistry : Research indicates that this compound can facilitate nitrene-extrusion reactions when coordinated with iron carbonyl complexes, leading to the formation of nitrogen-containing heterocycles .
Data Table: Reactivity of this compound Derivatives
Reaction Type | Conditions | Products | Reference |
---|---|---|---|
Diels-Alder | Pyrrole + Alkene | Bicyclic adducts | |
Nitrene Extrusion | Iron carbonyl complexes | Nitrogen heterocycles | |
Michael Addition | Thiols | Thiol adducts |
Medicinal Chemistry
The potential medicinal applications of this compound derivatives are notable:
- Thiol-Reactive Cleavable Linkers : These compounds have been explored as thiol-reactive linkers in drug delivery systems. They undergo Michael addition with thiols followed by retro-Diels–Alder cleavage, allowing controlled release of therapeutic agents .
- Anticancer Agents : Some derivatives have shown promise as anticancer agents due to their ability to form reactive intermediates that can interact with biological targets.
Case Study: Thiol-Reactive Linkers
A study demonstrated the use of azanorbornadienes as thiol-responsive cleavable linkages. The rates of retro-Diels-Alder cleavage varied significantly based on substituents at the nitrogen center, indicating the tunability of these compounds for specific applications in drug delivery systems .
Material Science
Beyond organic synthesis and medicinal chemistry, this compound has applications in material science:
- Polymer Chemistry : The compound can be used to synthesize functionalized polymers through its reactive sites, which can be tailored for specific properties.
Data Table: Applications in Material Science
Application | Description | Potential Benefits |
---|---|---|
Polymer Synthesis | Functionalized polymers via azanorbornadiene derivatives | Customizable properties for specific applications |
Q & A
Basic Research Questions
Q. What are the common synthetic pathways for 7-azanorbornadiene, and how do experimental conditions influence yield and purity?
- Methodological Answer: Synthesis typically involves [1,3]-dipolar cycloaddition or Diels-Alder reactions, with precise control of temperature, solvent polarity, and catalyst selection critical for optimizing yield. For example, the synthesis of this compound derivatives requires anhydrous conditions and inert atmospheres to prevent side reactions. Characterization via 1H-NMR and GC-MS is essential to confirm purity, while column chromatography is often employed for isolation .
Q. How do photoelectron (PE) spectra aid in analyzing the electronic structure of this compound?
- Methodological Answer: He(I) PE spectra provide insights into the energy levels of molecular orbitals, particularly the nitrogen lone pair and π-bond interactions. The split between the first two PE bands (e.g., 0.98 eV in this compound) correlates with through-space interactions between the nitrogen lone pair and adjacent π systems. Computational methods like STO-3G fragment orbital analysis are used to interpret spectral data and assign orbital orientations .
Advanced Research Questions
Q. What experimental and computational strategies resolve contradictions in orbital interaction models for this compound isomers?
- Methodological Answer: Discrepancies between syn- and anti-isomer stability can be addressed by combining PE spectroscopy with ab initio calculations. For instance, this compound’s syn-isomer exhibits stronger lone-pair/π interactions (FantiΨnπ=−0.71eV) compared to the anti-isomer (FantiΨnπ=+0.09eV). Hybrid QM/MM simulations further validate these interactions by modeling geometric distortions and electron density distributions .
Q. How does structural isomerism in this compound derivatives impact their reactivity in Diels-Alder reactions?
- Methodological Answer: Isomer-specific reactivity is studied using kinetic assays and DFT calculations. For example, syn-7-azanorbornadiene exhibits higher electrophilicity due to enhanced n→π* conjugation, accelerating cycloaddition rates. Transition-state analysis (IRC calculations) and Hammett plots quantify electronic effects, while X-ray crystallography confirms stereochemical outcomes .
Q. What methodologies reconcile discrepancies between experimental and computational data on this compound’s electronic properties?
- Methodological Answer: Systematic validation involves benchmarking computational methods (e.g., CCSD(T) vs. DFT) against experimental PE spectra and dipole moment measurements. For instance, STO-3G-level fragment orbital analysis accurately predicts the 1.26 eV band separation in syn-7-azanorbornadiene, aligning with the observed 0.98 eV split. Error analysis (e.g., basis set superposition errors) refines model accuracy .
Q. How can researchers address challenges in characterizing transient intermediates during this compound functionalization?
- Methodological Answer: Time-resolved spectroscopy (e.g., ultrafast UV-Vis or TRIR) coupled with cryogenic trapping isolates intermediates like aziridinium ions. Quenching experiments with nucleophiles (e.g., NaN3) and LC-MS analysis identify transient species. Computational transient state modeling (e.g., TD-DFT) predicts intermediate lifetimes and reaction pathways .
Q. Methodological Considerations
Q. What statistical approaches are recommended for analyzing contradictory data in this compound studies?
- Methodological Answer: Bayesian inference or multivariate regression models quantify uncertainties in spectral or kinetic data. For example, Monte Carlo simulations assess the impact of synthetic variability (e.g., temperature fluctuations) on reaction yield. Sensitivity analysis identifies dominant variables, while Bland-Altman plots validate reproducibility across experimental replicates .
Q. How should researchers design experiments to minimize artifacts in this compound’s spectral data?
- Methodological Answer: Control experiments (e.g., solvent blanks and deuterated analogs) isolate solvent and impurity effects. Baseline correction algorithms (e.g., asymmetric least squares) enhance PE spectral resolution. Collaborative inter-laboratory studies using standardized protocols (e.g., ASTM guidelines) ensure data comparability .
Q. Data Reporting and Reproducibility
Q. What guidelines ensure transparent reporting of this compound synthesis and characterization?
- Methodological Answer: Follow the Beilstein Journal of Organic Chemistry’s standards: report detailed synthetic protocols (reagents, stoichiometry, purification steps) in the main text, with raw spectral data in supplementary materials. Use IUPAC nomenclature and include error margins for yield and purity metrics. Reference previously published procedures for common steps .
Q. How can researchers enhance reproducibility in computational studies of this compound?
- Methodological Answer: Provide full computational details: software versions, basis sets, convergence criteria, and input files (as supplementary data). Publish Cartesian coordinates of optimized geometries and vibrational frequencies. Use platforms like Zenodo to archive datasets and enable independent validation .
Comparison with Similar Compounds
Comparison with Structurally Similar Compounds
7-Azabenzonorbornadiene
- Structure: Aromatic benzene ring fused to the norbornadiene system.
- Reactivity : Undergoes iEDDA with tetrazines to yield isoindoles, but the products are unstable due to dienic character .
- Kinetics : Reaction with 3,6-di-(2-pyridyl)-s-tetrazine (DPTz) proceeds at k ≈ 0.025 M⁻¹s⁻¹ (37°C), similar to 7-ANBD .
- Key Difference: Unlike 7-ANBD, azabenzonorbornadienes lack a non-aromatic bicyclic backbone, reducing strain energy and limiting applications in stable bioconjugation .
7-Oxabenzonorbornadiene
- Structure : Oxygen atom replaces nitrogen at the bridgehead.
- Reactivity : Forms isobenzofurans upon reaction with tetrazines. These products are more stable than isoindoles but less versatile in bioconjugation due to oxygen’s lower nucleophilicity .
- Activation Barrier : ΔG‡ for iEDDA with DPTz is ~21 kcal/mol, comparable to 7-ANBD .
7-Azanorbornene
- Structure : Partially saturated analog of 7-ANBD with one double bond.
- Reactivity : Less strained than 7-ANBD (ΔG‡ for iEDDA ≈ 24 kcal/mol), leading to slower reaction kinetics (k ≈ 0.015 M⁻¹s⁻¹) .
- Applications : Primarily used in radical cascades for asymmetric synthesis, as demonstrated by Hodgson et al. .
Comparative Data Table
Functional Comparisons
Transition Metal Interactions
- 7-Azanorbornene: Forms stable complexes with carbonyliron, enabling applications in hindered rotation studies .
Preparation Methods
Lewis Acid-Catalyzed Diels–Alder Reactions
Aluminum Chloride (AlCl₃)-Mediated Synthesis
The AlCl₃-promoted reaction of 1-carbomethoxypyrrole with dimethyl acetylenedicarboxylate (DMAD) is a cornerstone method for synthesizing 7-azanorbornadiene derivatives. This approach, first optimized by Bansal et al., achieves near-quantitative yields (90–95%) under mild conditions (40°C, 1 hour in CH₂Cl₂) . The Lewis acid facilitates a stereospecific cis-addition of the pyrrole to DMAD, forming 2,3,7-tricarbomethoxy-7-azanorbornadiene as the primary product. Competing pathways yielding maleate or fumarate adducts are suppressed at low temperatures (~25°C), ensuring selectivity .
Mechanistic Insight : AlCl₃ activates the acetylene via coordination, lowering the activation energy for the [4+2] cycloaddition. The reaction proceeds through a polar transition state, as evidenced by linear free energy relationships in analogous systems . Post-reaction aqueous workup removes AlCl₃, simplifying purification.
Role of Reaction Conditions on Yield and Selectivity
Variations in solvent, temperature, and stoichiometry significantly impact outcomes:
Parameter | Optimal Value | Effect on Yield/Selectivity |
---|---|---|
Temperature | 40°C | Maximizes this compound yield |
AlCl₃ Concentration | 0.05 M | Prevents over-coordination |
Solvent | CH₂Cl₂ | Enhances Lewis acid activity |
Elevating temperatures beyond 60°C promotes retro-Diels–Alder decomposition, while excess AlCl₃ leads to side products like dimethyl 1-carbomethoxy-2-pyrrolyl maleate .
Thermal Cycloaddition Approaches
High-Temperature Synthesis and Limitations
Early methods by Prinzbach et al. relied on thermal activation, heating N-substituted pyrroles (e.g., N-benzylpyrrole) with DMAD at 105–142°C . These conditions, however, suffer from reversibility and retro-Diels–Alder side reactions, capping yields at 30–45%. For example, N-carbomethoxypyrrole and DMAD heated at 142°C for 48 hours yield only 40% this compound, with significant decomposition to pyrrole and acetylene .
Comparative Efficiency :
Method | Yield (%) | Temperature (°C) | Time (h) |
---|---|---|---|
AlCl₃-Catalyzed | 90–95 | 40 | 1 |
Thermal | 30–45 | 105–142 | 24–48 |
The thermal method remains relevant for substrates incompatible with Lewis acids but is largely supplanted by catalytic approaches.
Inverse Electron Demand Diels–Alder (iEDDA) Strategies
Tetrazine-Based Cycloaddition and Fragmentation
A modern tandem iEDDA/retro-Diels–Alder (rDA) strategy enables access to β-substituted 7-azanorbornadienes. Reacting this compound derivatives with electron-deficient tetrazines (e.g., 3,6-di(2-pyridyl)-1,2,4,5-tetrazine) triggers a sequence of cycloaddition and two rDA steps, yielding functionalized pyrroles . This method accommodates diverse substituents, including sulfonates and esters, with yields ranging from 50–80% .
Example Reaction :
$$
\text{this compound} + \text{Tetrazine} \xrightarrow{\text{iEDDA}} \text{Cycloadduct} \xrightarrow{\text{rDA}} \beta\text{-Substituted Pyrrole}
$$
One-Pot Synthesis from Alkyne Precursors
A streamlined one-pot protocol starts with alkynes (e.g., ethynyl tolyl sulfone) and pyrroles, generating this compound intermediates in situ. Subsequent iEDDA/rDA reactions afford 29 distinct heterocycles, demonstrating broad substrate tolerance . For instance, N-(p-trifluoromethylphenyl)pyrrole reacts with isopentylnitrile and o-aminobenzoic acid in THF to yield this compound derivatives in 61% yield after column chromatography .
Comparative Analysis of Synthetic Methods
Yield and Efficiency Considerations
Method | Advantages | Limitations |
---|---|---|
AlCl₃-Catalyzed | High yield, mild conditions | Sensitive to moisture |
Thermal | No catalyst required | Low yield, side reactions |
iEDDA/rDA | Functional group diversity | Requires specialized tetrazines |
Functional Group Compatibility
-
Electron-Withdrawing Groups (EWGs) : N-Sulfonyl and N-acyl pyrroles react efficiently in AlCl₃-mediated reactions but exhibit reduced stability toward rDA cleavage .
-
Bulky Substituents : N-aryl groups (e.g., p-tolyl) hinder iEDDA reactivity due to steric effects .
Stability and Post-Synthetic Modifications
7-Azanorbornadienes undergo diverse transformations:
-
Photochemical Rearrangement : Irradiation at 310 nm converts 2,3,7-tricarbomethoxy-7-azanorbornadiene to 3-azaquadricyclane, which thermally rearranges to azepines .
-
Iron Complexation : Tetracarbonyliron complexes form via coordination to the norbornadiene framework, enabling mechanistic studies of decomposition pathways .
Stability Trends :
Properties
CAS No. |
7092-27-5 |
---|---|
Molecular Formula |
C6H7N |
Molecular Weight |
93.13 g/mol |
IUPAC Name |
7-azabicyclo[2.2.1]hepta-2,5-diene |
InChI |
InChI=1S/C6H7N/c1-2-6-4-3-5(1)7-6/h1-7H |
InChI Key |
JQBWSORHWJAIBL-UHFFFAOYSA-N |
Canonical SMILES |
C1=CC2C=CC1N2 |
Origin of Product |
United States |
Disclaimer and Information on In-Vitro Research Products
Please be aware that all articles and product information presented on BenchChem are intended solely for informational purposes. The products available for purchase on BenchChem are specifically designed for in-vitro studies, which are conducted outside of living organisms. In-vitro studies, derived from the Latin term "in glass," involve experiments performed in controlled laboratory settings using cells or tissues. It is important to note that these products are not categorized as medicines or drugs, and they have not received approval from the FDA for the prevention, treatment, or cure of any medical condition, ailment, or disease. We must emphasize that any form of bodily introduction of these products into humans or animals is strictly prohibited by law. It is essential to adhere to these guidelines to ensure compliance with legal and ethical standards in research and experimentation.