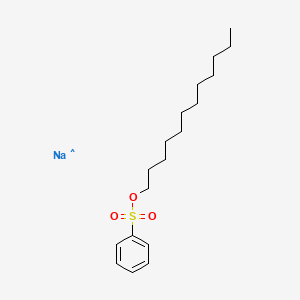
Sodium 4-(1-ethyldecyl)benzenesulfonate
Overview
Description
Sodium 4-(1-ethyldecyl)benzenesulfonate is a widely used anionic surfactant with the chemical formula C18H29NaO3S and a molecular weight of 348.48 . It is commonly found in household cleaning products, industrial applications, and scientific research due to its excellent wetting, dispersion, decontamination, and antibacterial properties . This compound is typically a white or yellowish powder or flake solid that is easily soluble in water, forming a translucent solution .
Preparation Methods
Synthetic Routes and Reaction Conditions: The synthesis of sodium dodecyl benzenesulfonate involves three main steps: alkylation, sulfonation, and neutralization .
Alkylation: Benzene reacts with dodecyl olefin to form dodecyl benzene.
Sulfonation: Dodecyl benzene is then sulfonated with sulfur trioxide (SO3) to produce dodecyl benzene sulfonic acid.
Neutralization: The sulfonic acid is neutralized with sodium hydroxide (NaOH) to yield sodium dodecyl benzenesulfonate.
Industrial Production Methods: In industrial settings, the production process involves the use of a continuous reactor system where benzene and dodecyl olefin are fed into an alkylation reactor. The resulting dodecyl benzene is then transferred to a sulfonation reactor where it reacts with sulfur trioxide. The sulfonated product is subsequently neutralized with sodium hydroxide in a neutralization reactor, and the final product is dried to obtain a free-flowing powder .
Chemical Reactions Analysis
Types of Reactions: Sodium 4-(1-ethyldecyl)benzenesulfonate undergoes various chemical reactions, including:
Oxidation: It can be oxidized to form sulfonic acids.
Reduction: It can be reduced to form corresponding hydrocarbons.
Substitution: It can undergo substitution reactions where the sulfonate group is replaced by other functional groups.
Common Reagents and Conditions:
Oxidation: Strong oxidizing agents like potassium permanganate (KMnO4) or hydrogen peroxide (H2O2) under acidic conditions.
Reduction: Reducing agents like lithium aluminum hydride (LiAlH4) or hydrogen gas (H2) in the presence of a catalyst.
Substitution: Reagents like sodium hydroxide (NaOH) or hydrochloric acid (HCl) under controlled conditions.
Major Products:
Oxidation: Sulfonic acids.
Reduction: Hydrocarbons.
Substitution: Various substituted benzenesulfonates.
Scientific Research Applications
Sodium 4-(1-ethyldecyl)benzenesulfonate has a wide range of applications in scientific research:
Chemistry: Used as a surfactant in the synthesis of nanoparticles and in the stabilization of dispersions.
Biology: Employed in the preparation of cell membranes and in protein extraction processes.
Medicine: Utilized in drug delivery systems and as an emulsifying agent in pharmaceutical formulations.
Industry: Widely used in the production of detergents, emulsifiers, and foaming agents.
Mechanism of Action
The mechanism of action of sodium dodecyl benzenesulfonate involves its ability to reduce surface tension and form micelles. The hydrophobic dodecyl chain interacts with non-polar substances, while the hydrophilic sulfonate group interacts with water, allowing it to effectively emulsify and disperse oils and other hydrophobic materials . This property is crucial in its role as a surfactant in various applications, including enhanced oil recovery and cleaning products .
Comparison with Similar Compounds
Sodium dodecyl sulfate (SDS): Another widely used anionic surfactant with similar properties but different structural characteristics.
Hexadecyltrimethylammonium bromide (CTAB): A cationic surfactant used in similar applications but with different ionic properties.
Properties
Molecular Formula |
C18H30NaO3S |
---|---|
Molecular Weight |
349.5 g/mol |
InChI |
InChI=1S/C18H30O3S.Na/c1-2-3-4-5-6-7-8-9-10-14-17-21-22(19,20)18-15-12-11-13-16-18;/h11-13,15-16H,2-10,14,17H2,1H3; |
InChI Key |
GVGUFUZHNYFZLC-UHFFFAOYSA-N |
Canonical SMILES |
CCCCCCCCCCCCOS(=O)(=O)C1=CC=CC=C1.[Na] |
melting_point |
>300 °C |
physical_description |
WHITE-TO-YELLOW SOLID IN VARIOUS FORMS. |
solubility |
Solubility in water, g/100ml at 25 °C: 20 |
Origin of Product |
United States |
Synthesis routes and methods I
Procedure details
Synthesis routes and methods II
Procedure details
Synthesis routes and methods III
Procedure details
Synthesis routes and methods IV
Procedure details
Disclaimer and Information on In-Vitro Research Products
Please be aware that all articles and product information presented on BenchChem are intended solely for informational purposes. The products available for purchase on BenchChem are specifically designed for in-vitro studies, which are conducted outside of living organisms. In-vitro studies, derived from the Latin term "in glass," involve experiments performed in controlled laboratory settings using cells or tissues. It is important to note that these products are not categorized as medicines or drugs, and they have not received approval from the FDA for the prevention, treatment, or cure of any medical condition, ailment, or disease. We must emphasize that any form of bodily introduction of these products into humans or animals is strictly prohibited by law. It is essential to adhere to these guidelines to ensure compliance with legal and ethical standards in research and experimentation.