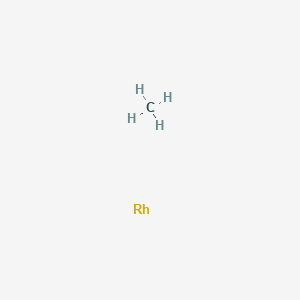
Rhodium carbon
- Click on QUICK INQUIRY to receive a quote from our team of experts.
- With the quality product at a COMPETITIVE price, you can focus more on your research.
Overview
Description
Rhodium carbon refers to rhodium-based catalysts, often supported on carbon or integrated into carbon-containing matrices, widely used in industrial and synthetic chemistry. These catalysts exhibit exceptional activity in hydroformylation, carbonylation, CO₂ utilization, and hydrocarbon reforming processes . Key properties include high tolerance to carbon monoxide (CO) poisoning, resistance to carbon deposition, and stability under harsh reaction conditions. For example, nanostructured rhodium films demonstrate enhanced CO electrooxidation due to their porous morphology . Rhodium's versatility is further highlighted in its ability to form active complexes with ligands such as phosphines, carbenes, and nitrogen-doped supports, enabling tailored reactivity and selectivity .
Chemical Reactions Analysis
C–H Bond Activation and Carboxylation
Rhodium(I) complexes catalyze the carboxylation of aromatic C–H bonds using CO₂, forming aryl carboxylic acids. Key mechanistic insights include:
Table 1: Mechanistic Features of Rh(I)-Catalyzed Carboxylation
Critical Factors :
-
Stirring rate and vessel geometry influence CO₂ solubility, favoring C–H activation over side reactions .
-
Catalytic conditions enable turnover despite stoichiometric carboxylation being faster .
N–H Insertion of Carbenes
Rh₂(OAc)₄ catalyzes solvent-free N–H insertions under mechanochemical conditions (ball milling):
Oxidative Carbonylation of Arenes
Rhodium catalyzes the direct conversion of aromatic C–H bonds to esters using CO and Oxone as an oxidant:
Table 3: Substrate Scope for Ester Formation
Mechanism : Oxone regenerates the active Rh species, enabling catalytic turnover .
Enantioselective Alder–Ene Reactions
Rhodium(I) hydride species mediate asymmetric couplings between alkenes and alkynes:
Table 4: Key Findings from Deuterium-Labeling Studies
Catalytic Cycle :
-
Oxidative addition of PhMe₂CCO₂H forms RhH species.
-
Alkyne insertion generates vinylrhodium intermediates.
-
Coordination to alkene enables enantioselective C–C bond formation .
Transfer Arylation via C–C Bond Cleavage
Rhodium-NHC complexes catalyze reversible C–C bond activation in alcohols:
Table 5: Mechanistic Insights from DFT Studies
Step | Energy Barrier (kcal/mol) | Key Intermediate |
---|---|---|
β-Carbon Elimination | 25.6 (rate-limiting) | Rh–alkoxide with η²-NHC coordination . |
Ketone Exchange | 18.3 | Rh–aryl species . |
Resting State : Chloride-bound Rh complex ([Rh(IPr*OMe)Cl]) dominates off-cycle .
Cycloaddition Reactions with Alkynes/CO
Rh(III) catalysts enable (5+2) and (5+1) cycloadditions for benzoxepine and coumarin synthesis:
Table 6: Computational Insights (M06-2X Method)
Reaction Type | Key Step | Activation Energy |
---|---|---|
(5+2) with Ethyne | Alkyne insertion into Rh–C bond | 22.1 kcal/mol . |
(5+1) with CO | CO insertion into Rh–O bond | 18.7 kcal/mol . |
Regioselectivity : Alkyne insertion favors Rh–C over Rh–O due to lower energy barriers .
Q & A
Basic Research Questions
Q. How can researchers optimize the synthesis of rhodium carbon catalysts to ensure consistent catalytic activity?
- Methodological Answer : Synthesis optimization involves systematic variation of parameters such as carbon support pre-treatment (e.g., acid washing to remove impurities), rhodium precursor selection (e.g., RhCl₃ vs. Rh(NO₃)₃), and reduction conditions (temperature, H₂ flow rate). Characterization via TEM and XRD should confirm Rh nanoparticle size (ideally <5 nm) and dispersion. For reproducibility, document pre-treatment protocols and use standardized reduction profiles .
Q. What characterization techniques are essential for verifying the structural integrity of Rh/C catalysts?
- Methodological Answer : Key techniques include:
- X-ray Photoelectron Spectroscopy (XPS) : To confirm Rh oxidation states (Rh⁰ vs. Rh³⁺) and carbon functionalization.
- Brunauer-Emmett-Teller (BET) Analysis : To assess surface area (>500 m²/g for activated carbon supports).
- Transmission Electron Microscopy (TEM) : To quantify Rh nanoparticle size distribution and agglomeration.
Cross-validate results with CO chemisorption for active site quantification .
Q. How can researchers address inconsistencies in catalytic performance data across studies using Rh/C?
- Methodological Answer : Discrepancies often arise from differences in reaction conditions (e.g., pH, temperature gradients) or support material purity. Standardize testing protocols (e.g., fixed-bed reactor setups) and report full experimental metadata (e.g., gas hourly space velocity, solvent purity). Use control experiments with reference catalysts (e.g., Pt/C) to benchmark activity .
Advanced Research Questions
Q. What mechanisms underlie the deactivation of Rh/C catalysts under oxidative conditions, and how can they be mitigated?
- Methodological Answer : Deactivation pathways include Rh nanoparticle sintering (addressed via support stabilization with SiO₂ coatings) and carbon support oxidation (mitigated by using graphitized carbon). Advanced techniques like in-situ XAS (X-ray Absorption Spectroscopy) can monitor structural changes during operation. Post-reaction TGA-MS analysis identifies carbon corrosion products (e.g., CO₂ evolution at >400°C) .
Q. How can in-situ spectroscopic methods be applied to study reaction intermediates on Rh/C surfaces?
- Methodological Answer :
- Operando FTIR : Track adsorbed CO or NOx species during catalysis.
- XANES/EXAFS : Resolve Rh coordination changes during hydrogenation reactions.
- Raman Spectroscopy : Detect carbon defect sites influencing Rh anchoring.
Combine with DFT modeling to correlate spectral data with proposed mechanisms .
Q. What strategies resolve contradictions between theoretical predictions and experimental results in Rh/C systems?
- Methodological Answer : Discrepancies may stem from oversimplified models (e.g., ignoring solvent effects or support interactions). Refine computational models by:
- Including solvent molecules explicitly in DFT calculations.
- Simulating carbon support defects (e.g., edge sites, oxygen vacancies).
Validate with kinetic isotope effect (KIE) studies or microkinetic modeling .
Q. How can researchers design experiments to probe the role of carbon support morphology in Rh/C activity?
- Methodological Answer : Use controlled synthesis of carbon supports (e.g., mesoporous vs. microporous carbons) and compare Rh dispersion and turnover frequency (TOF). Perform accelerated aging tests (e.g., cyclic voltammetry in acidic media) to assess stability. Correlate with BET surface area and pore size distribution data .
Q. Data and Reproducibility
Q. What steps ensure reproducibility in Rh/C catalyst synthesis and testing?
- Methodological Answer :
- Documentation : Provide detailed synthesis protocols (e.g., precursor concentrations, stirring durations) in supplementary materials.
- Reference Materials : Include characterization data for commercial Rh/C (e.g., Johnson Matthey) as benchmarks.
- Statistical Reporting : Use error bars for TOF measurements (minimum triplicate runs) and report confidence intervals .
Q. How should researchers assess the quality of literature data on Rh/C for meta-analysis?
- Methodological Answer : Apply criteria such as:
- Experimental Transparency : Availability of raw data (e.g., XRD patterns, kinetic plots).
- Control Experiments : Use of inert supports (e.g., Al₂O₃) to isolate Rh effects.
- Data Consistency : Cross-check TOF values with similar reaction conditions (e.g., 1 atm H₂, 25°C) .
Q. Emerging Research Directions
Q. What novel applications of Rh/C are being explored beyond traditional catalysis?
- Methodological Answer : Investigate Rh/C in:
- Electrocatalysis : CO₂ reduction to formic acid (FE >80% at -0.8 V vs. RHE).
- Biomedicine : H₂ production for antioxidant therapies (validate via in-vitro ROS scavenging assays).
- Photocatalysis : Couple with TiO₂ for UV-driven H₂ evolution (optimize Rh loading <1 wt%) .
Comparison with Similar Compounds
Nickel-Based Catalysts
Nickel catalysts are cost-effective alternatives but suffer from significant carbon accumulation during hydrocarbon reforming. In ethylene-rich environments (50,000 vol-ppm), nickel catalysts accumulate twice as much carbon as rhodium catalysts. Increasing the temperature to 960°C further doubles carbon deposition on nickel, while rhodium shows minimal sensitivity to temperature variations . Over prolonged reaction times (up to 144 hours), nickel catalysts exhibit linear carbon accumulation, whereas rhodium maintains stable performance with negligible carbon growth . This stark difference underscores rhodium's superior resistance to deactivation.
Iridium Catalysts
Iridium nanoparticles outperform rhodium in steam methane reforming (SMR), demonstrating higher activity and resistance to carbon deposition and sintering . However, iridium's scarcity and cost limit its industrial adoption compared to rhodium, which remains preferred for large-scale hydroformylation and carbonylation processes .
Cobalt Catalysts
Cobalt-based catalysts, historically used in hydroformylation, require high pressures (200–300 bar) and temperatures. Rhodium operates efficiently under milder conditions (10–50 bar, 80–120°C) with higher selectivity for linear aldehydes, making it the industry standard .
Phosphine-Containing vs. Phosphine-Free Rhodium Catalysts
Traditional rhodium catalysts employ phosphine ligands (e.g., RhCl(PPh₃)₃) to stabilize active sites. Recent advancements in phosphine-free rhodium catalysts, such as those supported on nitrogen-doped silica, show comparable hydroformylation activity while reducing environmental and cost burdens associated with phosphine ligands .
Single-Atom Rhodium vs. Complexes
Iron-Based Catalysts
Iron(II) catalysts have emerged as sustainable alternatives for alkene hydroformylation under mild conditions, but their activity and selectivity remain inferior to rhodium systems .
Data Tables
Table 1: Performance of Rhodium, Nickel, and Iridium in Catalytic Reforming
Property | Rhodium | Nickel | Iridium |
---|---|---|---|
Carbon Accumulation* | Low (0.5–1.0 g/gcat) | High (2.0–4.0 g/gcat) | Very Low (<0.5 g/gcat) |
Temperature Sensitivity | Low | High | Moderate |
Deactivation Resistance | High | Low | Very High |
Cost | High | Low | Very High |
*At 50,000 vol-ppm ethylene, 960°C .
Table 2: Comparison of Rhodium Catalyst Types in Hydroformylation
Catalyst Type | Activity (TOF, h⁻¹) | Selectivity (n/iso) | Conditions |
---|---|---|---|
RhCl(PPh₃)₃ | 500–800 | 3:1 | 80°C, 20 bar |
Phosphine-Free Rh/SiO₂ | 450–700 | 2.5:1 | 100°C, 30 bar |
Single-Atom Rh | 600–900 | 3.2:1 | 90°C, 15 bar |
TOF = Turnover frequency; n/iso = Linear-to-branched product ratio .
Key Research Findings
- Carbon Deposition : Rhodium catalysts exhibit 50% lower carbon accumulation than nickel in ethylene reforming, with stability maintained over 144 hours .
- CO Tolerance: Nanostructured rhodium films (50,000 laser pulses) show high porosity, enabling efficient CO electrooxidation at low overpotentials .
- Hydroformylation Efficiency: Phosphine-free rhodium catalysts achieve turnover frequencies (TOFs) of 450–700 h⁻¹, rivaling traditional phosphine-liganded systems .
- Structural Insights : Rhodium-carbon bonds in carbene complexes are best described as double bonds, contrasting with gold's single-bond behavior .
Properties
Molecular Formula |
CH4Rh |
---|---|
Molecular Weight |
118.948 g/mol |
IUPAC Name |
methane;rhodium |
InChI |
InChI=1S/CH4.Rh/h1H4; |
InChI Key |
RPNNPZHFJPXFQS-UHFFFAOYSA-N |
Canonical SMILES |
C.[Rh] |
Origin of Product |
United States |
Disclaimer and Information on In-Vitro Research Products
Please be aware that all articles and product information presented on BenchChem are intended solely for informational purposes. The products available for purchase on BenchChem are specifically designed for in-vitro studies, which are conducted outside of living organisms. In-vitro studies, derived from the Latin term "in glass," involve experiments performed in controlled laboratory settings using cells or tissues. It is important to note that these products are not categorized as medicines or drugs, and they have not received approval from the FDA for the prevention, treatment, or cure of any medical condition, ailment, or disease. We must emphasize that any form of bodily introduction of these products into humans or animals is strictly prohibited by law. It is essential to adhere to these guidelines to ensure compliance with legal and ethical standards in research and experimentation.