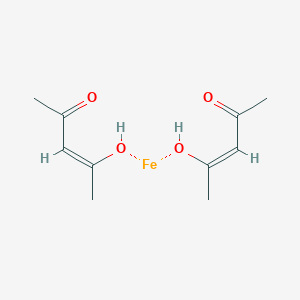
Ferrous acetylacetonate
Overview
Description
Ferrous acetylacetonate (CAS 14024-17-0), with the chemical formula C₁₀H₁₄FeO₄, is an organometallic compound where iron(II) coordinates with two acetylacetonate ligands. This dark brown crystalline solid is soluble in organic solvents and exhibits paramagnetic behavior due to the Fe²⁺ center . It is commercially significant, with applications spanning catalysis, polymer stabilization, and materials science. Market reports highlight its use in niche industrial processes, though detailed technical data on its synthesis and reactivity remain less publicly disseminated compared to other transition metal acetylacetonates .
Preparation Methods
Synthetic Routes and Reaction Conditions: The preparation of ferrous acetylacetonate typically involves the reaction of iron(II) salts with acetylacetone in the presence of a base. The general reaction can be represented as follows: [ \text{Fe}^{2+} + 2 \text{Hacac} \rightarrow \text{Fe(acac)}_2 + 2 \text{H}^+ ] In this reaction, the iron(II) ion reacts with acetylacetone (Hacac) to form this compound (Fe(acac)2) and hydrogen ions. The addition of a base helps to deprotonate the acetylacetone, shifting the equilibrium towards the formation of the complex .
Industrial Production Methods: Industrial production of this compound follows similar principles but often involves more controlled conditions to ensure high purity and yield. The process may include steps such as recrystallization from hot petroleum ether to obtain pure crystalline samples .
Chemical Reactions Analysis
Catalytic Intramolecular C–H Alkoxylation
Fe(acac)₂ serves as an efficient catalyst for synthesizing O-heterocycles through radical-mediated intramolecular C–H alkoxylation. In reactions involving α-diazo-β-ketoesters, Fe(acac)₂ promotes cyclization via a proposed mechanism involving:
-
Substrate binding to the Fe center through ketoester functionalities.
-
Electron transfer from Fe(II) to the diazo group, generating a Fe(III)-stabilized vinyl carboradical and releasing N₂.
-
1,5-Hydrogen atom transfer (HAT) to form an alkyl carboradical.
-
Cyclization via oxygen rebound to yield (Z)-2-alkylidene dihydrofurans .
Key data from catalytic studies
Substrate Type | Reaction Time | Temperature | Yield (%) |
---|---|---|---|
Tertiary C–H | 3 h | 25°C | 70 |
Benzylic C–H | 20 min | 60°C | 85 |
Secondary C–H | 20 min | 60°C | 78 |
The reaction shows selectivity for tertiary C–H bonds due to lower activation barriers (~2.9 kcal/mol for tertiary vs. ~5.4 kcal/mol for secondary) .
Redox and Ligand Exchange Reactions
Fe(acac)₂ participates in ligand exchange and redox processes in acetonitrile, as demonstrated in electrochemical studies:
-
Reduction of Fe(acac)₃ : Fe(acac)₂ forms via electron transfer, followed by ligand exchange with unreacted Fe(acac)₃ to produce Fe²⁺ and Fe(acac)₃ .
-
Oxidation of Fe(acac)₂ : Generates Fe(acac)₂⁺, which undergoes further ligand redistribution .
Process | Potential (V vs. SCE) | Key Products |
---|---|---|
Fe(acac)₃ reduction | -0.8 to +1.0 | Fe(acac)₂, Fe²⁺ |
Fe(acac)₂ oxidation | +0.4 to +1.0 | Fe(acac)₂⁺, Fe(acac)₃ |
The ligand exchange kinetics are influenced by solvent polarity and supporting electrolytes .
Stability and Reactivity Considerations
-
Moisture sensitivity : Rapid hydrolysis in aqueous environments limits its use in protic solvents .
-
Radical quenching : Adventitious hydrogen atom donors (e.g., cyclohexadiene) deactivate Fe(acac)₂ by trapping reactive intermediates .
-
Ligand redistribution : Dynamic equilibria between Fe(acac)₂, Fe(acac)₃, and Fe²⁺ occur in solution, complicating mechanistic studies .
Comparative Reactivity with Fe(acac)₃
Property | Fe(acac)₂ | Fe(acac)₃ |
---|---|---|
Oxidation state | +2 | +3 |
Magnetic moment | 4.9 μ<sub>B</sub> | 5.9 μ<sub>B</sub> |
Catalytic role | Radical initiation | Lewis acid catalysis |
Thermal stability | Lower (decomposes <210°C) | Higher (stable to 180°C) |
Scientific Research Applications
Catalytic Applications
Ferrous acetylacetonate is widely recognized for its role as a catalyst in various organic reactions. Its chelating ability allows it to stabilize reactive intermediates, enhancing reaction efficiency.
Key Catalytic Uses :
- Hydrogenation Reactions : Acts as a catalyst in hydrogenation processes, facilitating the conversion of unsaturated compounds to saturated ones.
- Cross-Coupling Reactions : Utilized in coupling reactions such as Suzuki and Heck reactions, where it aids in the formation of carbon-carbon bonds .
- Synthesis of Nanostructures : Employed in the chemical vapor deposition (CVD) process for synthesizing iron-containing nanostructures, which have applications in electronics and magnetic materials .
Material Science Applications
The compound's properties make it suitable for various applications in material science, particularly in the synthesis of advanced materials.
Applications in Material Science :
- Thin Film Deposition : Used as a precursor for thin films in semiconductor manufacturing and coatings due to its volatility and stability under heat .
- Magnetic Materials : Its magnetic properties are exploited in the production of low-grade magnets and memory tapes .
Electrochemical Applications
This compound has been studied for its electrochemical properties, particularly in energy storage systems.
Electrochemical Applications :
- Redox Flow Batteries : The redox couple involving Fe(acac)₂ has been investigated for use in redox flow batteries, providing insights into its potential as an electrolyte component .
- Dye-Sensitized Solar Cells (DSSCs) : Research indicates that Fe(acac)₂ can be used as a redox mediator in p-type dye-sensitized solar cells, enhancing their efficiency .
Case Study 1: Catalytic Performance
A study demonstrated the effectiveness of this compound in catalyzing the synthesis of complex organic compounds through cross-coupling reactions. The reaction conditions were optimized to achieve high yields with minimal by-products .
Case Study 2: Electrochemical Properties
Research on the electrochemical behavior of Fe(acac)₂ revealed its potential use in energy storage systems. The compound showed favorable redox characteristics that could be harnessed for efficient charge transfer processes .
Mechanism of Action
The mechanism of action of ferrous acetylacetonate involves its ability to coordinate with other molecules through its iron center. The acetylacetonate ligands stabilize the iron(II) ion, allowing it to participate in various chemical reactions. The molecular targets and pathways involved depend on the specific application, such as catalysis or biological interactions .
Comparison with Similar Compounds
Comparison with Similar Compounds
The acetylacetonate ligand (acac) forms stable complexes with diverse metals, enabling tailored electronic and steric properties. Below, ferrous acetylacetonate is compared to analogous complexes in terms of structure, reactivity, and applications.
Zinc Acetylacetonate
- Structure/Properties : Zinc acetylacetonate (Zn(acac)₂) adopts a tetrahedral geometry. Unlike this compound, it is diamagnetic due to Zn²⁺’s filled d-orbitals.
- Applications: Widely used as a heat stabilizer in rigid PVC, it synergizes with β-diketones to inhibit thermal degradation .
- Catalytic Efficiency: Less effective in redox catalysis compared to iron analogs but excels in transesterification (47.8% phenol conversion) .
Titanium Acetylacetonate
- Reactivity : Titanium acetylacetonate (Ti(acac)₄) catalyzes transesterification via a six-membered ring intermediate, achieving >99.9% selectivity for diphenyl carbonate. This contrasts with this compound, which is less studied in such reactions .
- Thermal Stability : Decomposes at lower temperatures (~200°C) compared to this compound, limiting high-temperature applications .
Cobalt Acetylacetonate Derivatives
- Electronic Tuning : Modifying acac ligands with electron-withdrawing groups (e.g., -CF₃) in Co(acac)₂ increases CO stretching frequencies (IR spectroscopy), enhancing catalytic activity in Negishi coupling. This compound lacks analogous electronic tunability studies .
- Performance : Co(acac)₂ derivatives yield (E)-5-dodecene with higher efficiency (85% yield) than unmodified Fe(acac)₂ in cross-coupling reactions .
Calcium Acetylacetonate
- Industrial Use : Calcium acetylacetonate (Ca(acac)₂) stabilizes PVC and improves coating durability, similar to zinc analogs. However, its market growth is constrained by regulatory hurdles and competition from eco-friendly alternatives, challenges less emphasized for this compound .
- Thermal Stability : Outperforms this compound in polymer processing due to higher decomposition thresholds (>300°C) .
Iridium Acetylacetonate
- Applications : Ir(acac)₃ is used in hydrogenation and advanced material synthesis (e.g., coordination polymers). Its catalytic scope and thermal stability (melting point: 269–271°C) exceed this compound’s, albeit at higher costs .
Chromium and Aluminum Acetylacetonates
- Catalysis: Cr(acac)₃ and Al(acac)₃ catalyze organosilicon reactions, leveraging their Lewis acidity. This compound’s redox activity makes it more suited for oxidative processes .
Structural and Mechanistic Insights
- Coordination Geometry : this compound’s octahedral Fe²⁺ center contrasts with hexacoordinated Fe³⁺ in Fe(acac)₃, which shows a single Mössbauer absorption peak (isomer shift: 0.50 mm/s). Co³⁺ analogs exhibit charge stabilization post-K-capture, a feature unexplored in Fe²⁺ systems .
- Ligand Dissociation: In ferrimagnetic ZnFe₂O₄ synthesis, Fe(acac)₃ loses ligands at 90°C (89% dissociation), whereas Fe²⁺ derivatives like this compound likely exhibit slower kinetics, affecting nanocrystal growth .
Comparative Data Table
Q & A
Basic Research Questions
Q. What are the standard synthesis protocols for ferrous acetylacetonate, and how do reaction conditions influence product purity?
this compound is typically synthesized by reacting ferrous chloride (FeCl₂) with acetylacetone in the presence of a base (e.g., sodium acetate) under an inert atmosphere to prevent oxidation. The solvent (e.g., ethanol or water), temperature (often 50–80°C), and stoichiometric ratios are critical for achieving high purity. For example, excess acetylacetone ensures complete chelation of Fe²⁺, while inert conditions minimize oxidation to Fe³⁺ derivatives. Product purity can be assessed via elemental analysis and IR spectroscopy to confirm ligand coordination .
Q. Which spectroscopic and analytical techniques are most effective for characterizing this compound’s structure and purity?
- 1H-NMR with Evans Method : Determines magnetic susceptibility and unpaired electrons, critical for confirming the paramagnetic Fe²⁺ center. However, paramagnetic broadening complicates peak resolution, requiring careful data interpretation .
- IR Spectroscopy : Identifies characteristic C=O and C=C stretching frequencies (~1520–1600 cm⁻¹) of the acetylacetonate ligand.
- XRD : Confirms crystalline structure and phase purity.
- Elemental Analysis : Validates stoichiometry (C, H, Fe content). Cross-referencing these methods ensures structural fidelity .
Q. What safety protocols are essential when handling this compound in laboratory settings?
Use gloves, lab coats, and fume hoods to avoid skin contact and inhalation. Store in airtight containers under inert gas (e.g., N₂) to prevent oxidation. Waste should be segregated and treated as hazardous due to potential metal leaching. Protocols for analogous metal acetylacetonates (e.g., silver derivatives) provide a framework for safe handling .
Advanced Research Questions
Q. How can computational modeling (e.g., DFT) be validated against experimental thermodynamic data for this compound?
Density Functional Theory (DFT) calculations can predict thermodynamic properties like vapor pressure and Gibbs free energy. Validation involves comparing computed values with experimental data from thermogravimetric analysis (TGA) or vapor pressure measurements. For instance, discrepancies in Fe(aa)₃ vapor pressure curves (experimental vs. calculated) highlight the need to refine solvation or entropy parameters in models .
Q. What experimental strategies mitigate oxidation of this compound during handling, and how does oxidation impact subsequent reactivity?
Oxidation to Fe³⁺ species is minimized by:
- Conducting reactions under N₂/Ar.
- Using degassed solvents.
- Storing samples at low temperatures (-20°C). Oxidation alters redox activity, as seen in catalytic applications where Fe²⁺→Fe³⁺ shifts ligand exchange kinetics. Redox state verification via cyclic voltammetry or Mössbauer spectroscopy is critical for reproducibility .
Q. How does the coordination environment of this compound influence its catalytic activity in cross-coupling or polymerization reactions?
The octahedral geometry of Fe²⁺, with two bidentate acetylacetonate ligands and labile coordination sites, enables ligand substitution reactions. For example, in nanoparticle synthesis, labile ligands allow precursor dissociation, facilitating metal nucleation. Tuning ligand steric/electronic properties (e.g., substituting acetylacetone with fluorinated analogs) modulates catalytic turnover and selectivity .
Q. What role does this compound play in the synthesis of heterostructured materials, and how can synthesis parameters be optimized?
this compound serves as a precursor for Fe-based nanomaterials. In Fe/ZIF-8 composites, increasing precursor concentration enhances surface roughness of ZIF-8 nanocrystals, as shown in SEM/XRD studies. Optimization involves adjusting reaction time, temperature, and Fe(aa)₂:ZIF-8 ratios to balance structural integrity and catalytic performance .
Q. How should researchers address discrepancies in reported thermodynamic properties (e.g., decomposition temperatures) across studies?
Discrepancies often arise from differences in experimental setups (e.g., heating rates in TGA) or sample purity. Standardizing protocols (e.g., ISO-compliant TGA methods) and reporting detailed synthesis conditions (solvent, atmosphere) improve comparability. Cross-validation using multiple techniques (e.g., DSC for enthalpy changes) resolves ambiguities .
Q. Data Analysis and Contradiction Resolution
Q. How can conflicting reports on this compound’s stability in aqueous vs. organic solvents be reconciled?
Aqueous solutions of Fe(aa)₂ undergo rapid hydrolysis, forming colloidal Fe(OH)₂, whereas organic solvents (e.g., chloroform) stabilize the complex. Contradictions arise from solvent choice and pH; stability assays via UV-Vis spectroscopy under varied conditions (pH 3–10, solvents) clarify these dependencies .
Q. What methodologies resolve contradictions in ligand exchange kinetics reported for this compound?
Conflicting kinetic data may stem from differing ligand concentrations or detection methods (e.g., stopped-flow vs. conventional UV-Vis). Using pseudo-first-order conditions and global kinetic modeling (e.g., Eyring plots for activation parameters) standardizes comparisons. In situ techniques like FT-IR track ligand displacement in real time .
Application-Oriented Questions
Q. How is this compound utilized in energy storage systems, such as lithium-sulfur batteries?
Fe(aa)₂-derived FeS heterostructures enhance polysulfide redox kinetics by providing conductive pathways and adsorption sites. Electrochemical impedance spectroscopy (EIS) and galvanostatic cycling validate performance improvements, such as reduced charge transfer resistance .
Q. What advanced characterization techniques elucidate this compound’s role in photocatalytic applications?
X-ray absorption spectroscopy (XAS) probes Fe²⁺ coordination changes during photocatalysis. Transient absorption spectroscopy tracks charge carrier dynamics, linking ligand structure to photocatalytic efficiency (e.g., H₂ production rates) .
Properties
CAS No. |
14024-17-0 |
---|---|
Molecular Formula |
C10H16FeO4 |
Molecular Weight |
256.08 g/mol |
IUPAC Name |
(Z)-4-hydroxypent-3-en-2-one;iron |
InChI |
InChI=1S/2C5H8O2.Fe/c2*1-4(6)3-5(2)7;/h2*3,6H,1-2H3;/b2*4-3-; |
InChI Key |
LFKXWKGYHQXRQA-FDGPNNRMSA-N |
SMILES |
CC(=CC(=O)C)O.CC(=CC(=O)C)O.[Fe] |
Isomeric SMILES |
C/C(=C/C(=O)C)/O.C/C(=C/C(=O)C)/O.[Fe] |
Canonical SMILES |
CC(=CC(=O)C)O.CC(=CC(=O)C)O.[Fe] |
Key on ui other cas no. |
14024-17-0 |
Pictograms |
Irritant |
Origin of Product |
United States |
Retrosynthesis Analysis
AI-Powered Synthesis Planning: Our tool employs the Template_relevance Pistachio, Template_relevance Bkms_metabolic, Template_relevance Pistachio_ringbreaker, Template_relevance Reaxys, Template_relevance Reaxys_biocatalysis model, leveraging a vast database of chemical reactions to predict feasible synthetic routes.
One-Step Synthesis Focus: Specifically designed for one-step synthesis, it provides concise and direct routes for your target compounds, streamlining the synthesis process.
Accurate Predictions: Utilizing the extensive PISTACHIO, BKMS_METABOLIC, PISTACHIO_RINGBREAKER, REAXYS, REAXYS_BIOCATALYSIS database, our tool offers high-accuracy predictions, reflecting the latest in chemical research and data.
Strategy Settings
Precursor scoring | Relevance Heuristic |
---|---|
Min. plausibility | 0.01 |
Model | Template_relevance |
Template Set | Pistachio/Bkms_metabolic/Pistachio_ringbreaker/Reaxys/Reaxys_biocatalysis |
Top-N result to add to graph | 6 |
Feasible Synthetic Routes
Disclaimer and Information on In-Vitro Research Products
Please be aware that all articles and product information presented on BenchChem are intended solely for informational purposes. The products available for purchase on BenchChem are specifically designed for in-vitro studies, which are conducted outside of living organisms. In-vitro studies, derived from the Latin term "in glass," involve experiments performed in controlled laboratory settings using cells or tissues. It is important to note that these products are not categorized as medicines or drugs, and they have not received approval from the FDA for the prevention, treatment, or cure of any medical condition, ailment, or disease. We must emphasize that any form of bodily introduction of these products into humans or animals is strictly prohibited by law. It is essential to adhere to these guidelines to ensure compliance with legal and ethical standards in research and experimentation.