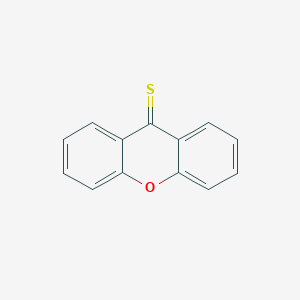
Xanthene-9-thione
Overview
Description
Xanthene-9-thione is an organic compound with the molecular formula C₁₃H₈OS. It is a sulfur-containing derivative of xanthene, characterized by the presence of a thioketone group at the 9th position of the xanthene ring system.
Mechanism of Action
Target of Action
Xanthene-9-thione, also known as 9H-Xanthene-9-thione, is a type of xanthone, a class of compounds known for their diverse biological activities . The primary targets of xanthones are often cancer cells, where they exhibit promising anti-cancer activities
Mode of Action
Xanthones in general have been shown to interact with their targets through various mechanisms, including apoptosis, autophagy, cell cycle arrest, and modulation of key signaling pathways such as pi3k/akt and mapk .
Biochemical Pathways
Xanthone biosynthesis in plants involves the shikimate and the acetate pathways, which originate in plastids and endoplasmic reticulum, respectively . The pathway continues following three alternative routes, two phenylalanine-dependent and one phenylalanine-independent
Result of Action
Xanthones in general have been shown to exhibit various biological activities, including α-glucosidase inhibition, anti-cancer activities, anti-alzheimer activities, and anti-inflammatory activities .
Action Environment
It’s worth noting that the photophysical properties of related compounds, such as thioxanthone, have been studied, and these compounds have been found to play a unique role in photochemistry .
Preparation Methods
Synthetic Routes and Reaction Conditions: Xanthene-9-thione can be synthesized through several methods. One common approach involves the reaction of xanthene with sulfur or sulfur-containing reagents under specific conditions. For instance, the reaction of xanthene with elemental sulfur in the presence of a catalyst such as iodine can yield this compound . Another method involves the use of thiourea as a sulfur source, where xanthene is treated with thiourea in the presence of an acid catalyst .
Industrial Production Methods: Industrial production of this compound typically involves large-scale synthesis using optimized reaction conditions to ensure high yield and purity. The process may include steps such as purification through recrystallization or chromatography to obtain the desired product .
Chemical Reactions Analysis
Types of Reactions: Xanthene-9-thione undergoes various chemical reactions, including:
Oxidation: It can be oxidized to form xanthene-9-sulfoxide or xanthene-9-sulfone under appropriate conditions.
Reduction: Reduction of this compound can yield xanthene or other reduced sulfur-containing derivatives.
Substitution: The thioketone group can participate in nucleophilic substitution reactions, where the sulfur atom is replaced by other nucleophiles.
Common Reagents and Conditions:
Oxidation: Common oxidizing agents include hydrogen peroxide and peracids.
Reduction: Reducing agents such as lithium aluminum hydride or sodium borohydride are often used.
Substitution: Nucleophiles like amines or thiols can be employed in substitution reactions.
Major Products:
Oxidation: Xanthene-9-sulfoxide, xanthene-9-sulfone.
Reduction: Xanthene, reduced sulfur derivatives.
Substitution: Various substituted xanthene derivatives depending on the nucleophile used.
Scientific Research Applications
Xanthene-9-thione has a wide range of applications in scientific research:
Comparison with Similar Compounds
Xanthene: The parent compound, lacking the thioketone group.
Xanthone: An oxygen-containing analog with a ketone group at the 9th position.
Thioxanthone: A sulfur-containing analog with a thioketone group at the 9th position but with different substitution patterns.
Uniqueness: Xanthene-9-thione is unique due to the presence of the thioketone group, which imparts distinct chemical reactivity and biological activities compared to its analogs. This makes it a valuable compound for various applications in research and industry .
Properties
IUPAC Name |
xanthene-9-thione | |
---|---|---|
Source | PubChem | |
URL | https://pubchem.ncbi.nlm.nih.gov | |
Description | Data deposited in or computed by PubChem | |
InChI |
InChI=1S/C13H8OS/c15-13-9-5-1-3-7-11(9)14-12-8-4-2-6-10(12)13/h1-8H | |
Source | PubChem | |
URL | https://pubchem.ncbi.nlm.nih.gov | |
Description | Data deposited in or computed by PubChem | |
InChI Key |
VKLOKHJKPPJQMM-UHFFFAOYSA-N | |
Source | PubChem | |
URL | https://pubchem.ncbi.nlm.nih.gov | |
Description | Data deposited in or computed by PubChem | |
Canonical SMILES |
C1=CC=C2C(=C1)C(=S)C3=CC=CC=C3O2 | |
Source | PubChem | |
URL | https://pubchem.ncbi.nlm.nih.gov | |
Description | Data deposited in or computed by PubChem | |
Molecular Formula |
C13H8OS | |
Source | PubChem | |
URL | https://pubchem.ncbi.nlm.nih.gov | |
Description | Data deposited in or computed by PubChem | |
DSSTOX Substance ID |
DTXSID60306237 | |
Record name | Xanthene-9-thione | |
Source | EPA DSSTox | |
URL | https://comptox.epa.gov/dashboard/DTXSID60306237 | |
Description | DSSTox provides a high quality public chemistry resource for supporting improved predictive toxicology. | |
Molecular Weight |
212.27 g/mol | |
Source | PubChem | |
URL | https://pubchem.ncbi.nlm.nih.gov | |
Description | Data deposited in or computed by PubChem | |
CAS No. |
492-21-7 | |
Record name | Xanthene-9-thione | |
Source | DTP/NCI | |
URL | https://dtp.cancer.gov/dtpstandard/servlet/dwindex?searchtype=NSC&outputformat=html&searchlist=174726 | |
Description | The NCI Development Therapeutics Program (DTP) provides services and resources to the academic and private-sector research communities worldwide to facilitate the discovery and development of new cancer therapeutic agents. | |
Explanation | Unless otherwise indicated, all text within NCI products is free of copyright and may be reused without our permission. Credit the National Cancer Institute as the source. | |
Record name | Xanthene-9-thione | |
Source | EPA DSSTox | |
URL | https://comptox.epa.gov/dashboard/DTXSID60306237 | |
Description | DSSTox provides a high quality public chemistry resource for supporting improved predictive toxicology. | |
Retrosynthesis Analysis
AI-Powered Synthesis Planning: Our tool employs the Template_relevance Pistachio, Template_relevance Bkms_metabolic, Template_relevance Pistachio_ringbreaker, Template_relevance Reaxys, Template_relevance Reaxys_biocatalysis model, leveraging a vast database of chemical reactions to predict feasible synthetic routes.
One-Step Synthesis Focus: Specifically designed for one-step synthesis, it provides concise and direct routes for your target compounds, streamlining the synthesis process.
Accurate Predictions: Utilizing the extensive PISTACHIO, BKMS_METABOLIC, PISTACHIO_RINGBREAKER, REAXYS, REAXYS_BIOCATALYSIS database, our tool offers high-accuracy predictions, reflecting the latest in chemical research and data.
Strategy Settings
Precursor scoring | Relevance Heuristic |
---|---|
Min. plausibility | 0.01 |
Model | Template_relevance |
Template Set | Pistachio/Bkms_metabolic/Pistachio_ringbreaker/Reaxys/Reaxys_biocatalysis |
Top-N result to add to graph | 6 |
Feasible Synthetic Routes
Q1: What is the molecular formula, weight, and key spectroscopic data for xanthene-9-thione?
A: this compound (also known as 9H-xanthene-9-thione) has the molecular formula C13H8OS and a molecular weight of 212.27 g/mol. While the provided research papers don't explicitly list all spectroscopic data, they utilize techniques like UV-Vis and ESR spectroscopy to study its reactivity and transient species. [, ] For a complete spectroscopic characterization, consult databases like NIST or SDBS.
Q2: How does this compound interact in photochemical reactions with allenes?
A: this compound, upon photoexcitation to its triplet state, reacts with allenes through a 1,4-biradical intermediate. This biradical, characterized by a short lifetime (90 ps), undergoes further reactions to form thiopyran derivatives. The product distribution (regioisomers E or F) is influenced by the substituent on the allene. [, ]
Q3: Can this compound act as a mediating agent in controlled radical polymerization?
A: Yes, this compound exhibits the capacity to mediate the controlled radical polymerization (CRP) of various monomers. Notably, it facilitates the CRP of vinyl chloride, a challenging monomer due to its high radical reactivity, enabling the synthesis of poly(vinyl chloride) (PVC) with controlled molecular weight and dispersity. []
Q4: How does this compound compare to other thioketones in terms of radical affinity?
A: Computational studies employing ab initio molecular orbital calculations have revealed that this compound possesses a relatively high radical affinity. This is attributed to the stability of the radical adduct formed upon radical addition to the C=S bond. While this compound exhibits good radical affinity, other thioketones like fluorine-9-thione have been computationally predicted to have even higher affinities, making them potentially more suitable for controlling radical polymerization. []
Q5: What is the mechanism behind the acetal formation from this compound and diols?
A: this compound undergoes desulfurization-condensation reactions with diols in the presence of silver(I) salts and a base like methylamine. This reaction proceeds through the interaction of the thioketone sulfur with silver(I), facilitating the nucleophilic attack of the diol on the carbonyl carbon, ultimately leading to acetal formation. []
Q6: How does the structure of this compound influence its reactivity towards diazo compounds?
A: this compound reacts with ethyl diazoacetate at elevated temperatures to yield an α,β-unsaturated ester as the sole product. This suggests a mechanism involving initial cycloaddition followed by rapid desulfurization. The reaction outcome underscores the influence of the xanthene backbone on the reactivity of the thiocarbonyl group. []
Q7: How do electron-donating substituents affect the spectral properties of this compound derivatives?
A: Introduction of electron-donating dimethylamino groups, as in 2,7-bis(dimethylamino)-9H-xanthene-9-thione, significantly influences the electronic properties and reactivity of the thioketone. This derivative exhibits enhanced electron density at the thiocarbonyl group, making it a more potent radical trap in polymerization reactions. []
Q8: Can this compound be used to synthesize other heterocyclic compounds?
A: Yes, this compound serves as a versatile building block in heterocyclic synthesis. For instance, it reacts with diphenylketene under photochemical conditions to yield a spiro-adduct containing a thietan-2-one ring. []
Q9: What are some alternative synthetic routes to xanthene-9-thiones?
A9: Several alternative synthetic approaches to this compound and its derivatives have been reported. These include the conversion of:
- 9-(Trimethylsilyl)-9-xanthene []
- 9-Xanthen-9-ones via 9,9-Dichloro-9-xanthenes []
- Bi-9-xanthen-9-ylidene Sulfide []
- 9-Alkylidene-9-xanthenes []
Q10: Are there any computational studies on the reactivity of this compound?
A: While the provided papers primarily focus on experimental investigations, computational chemistry plays a crucial role in understanding the reactivity of this compound and related thioketones. Ab initio molecular orbital calculations have been employed to determine radical affinities and predict potential applications in controlled radical polymerization. []
Q11: What analytical techniques are commonly used to characterize this compound and its derivatives?
A11: Various analytical techniques are employed to characterize this compound and its derivatives. These include:
Disclaimer and Information on In-Vitro Research Products
Please be aware that all articles and product information presented on BenchChem are intended solely for informational purposes. The products available for purchase on BenchChem are specifically designed for in-vitro studies, which are conducted outside of living organisms. In-vitro studies, derived from the Latin term "in glass," involve experiments performed in controlled laboratory settings using cells or tissues. It is important to note that these products are not categorized as medicines or drugs, and they have not received approval from the FDA for the prevention, treatment, or cure of any medical condition, ailment, or disease. We must emphasize that any form of bodily introduction of these products into humans or animals is strictly prohibited by law. It is essential to adhere to these guidelines to ensure compliance with legal and ethical standards in research and experimentation.