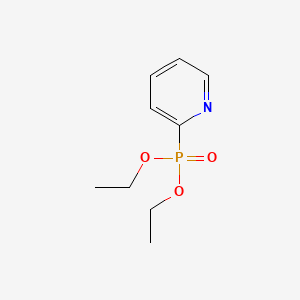
Diethyl 2-pyridylphosphonate
Overview
Description
Diethyl 2-pyridylphosphonate is an organophosphorus compound characterized by a pyridine ring substituted at the 2-position with a diethyl phosphonate group. This structural motif confers unique electronic and steric properties, making it a versatile catalyst and ligand in organic synthesis. A notable application is its role as a bidentate Lewis base catalyst in the hydrosilylation of aldehydes, where it activates HSiCl₃ to facilitate selective reduction . Its catalytic efficiency surpasses that of structurally related phosphonates, as demonstrated in comparative studies (see Section 2).
Preparation Methods
Synthetic Routes and Reaction Conditions: Diethyl 2-pyridylphosphonate can be synthesized through several methods. One common approach involves the reaction of 2-pyridylphosphonic dichloride with ethanol under basic conditions. The reaction typically proceeds as follows: [ \text{2-Pyridylphosphonic dichloride} + \text{Ethanol} \rightarrow \text{this compound} + \text{Hydrochloric acid} ]
Industrial Production Methods: Industrial production of this compound often employs similar synthetic routes but on a larger scale. The reaction conditions are optimized to ensure high yield and purity of the product. The use of continuous flow reactors and advanced purification techniques, such as distillation and crystallization, are common in industrial settings.
Chemical Reactions Analysis
Hirao Cross-Coupling Reactions
Diethyl 2-pyridylphosphonate participates in palladium-catalyzed Hirao cross-coupling reactions to form aryl and heteroaryl phosphonates. Key findings include:
-
Catalytic System : Using 1 mol% Pd(OAc)₂ with 1,1'-bis(diphenylphosphino)ferrocene (dppf) as a ligand in DMF at 110°C achieves optimal yields .
-
Substrate Scope :
-
Side Reactions : Dealkylation occurs with primary phosphites but is mitigated using N,N-diisopropylethylamine and diisopropyl phosphite, which reduce nucleophilic displacement .
Table 1: Hirao Cross-Coupling Reaction Outcomes
Substrate | Product | Yield (%) | Conditions |
---|---|---|---|
2-Chloropyrazine | Diethyl pyrazine-2-phosphonate | 67 | Pd(OAc)₂/dppf, DMF, 110°C |
2-Chloropyrimidine | Diethyl pyrimidine-2-phosphonate | 68 | Pd(OAc)₂/dppf, DMF, 110°C |
Hydrosilylation Catalysis
This compound acts as a bidentate Lewis base catalyst in the hydrosilylation of aldehydes using HSiCl₃. Highlights include:
-
Mechanism : Coordination of the pyridyl nitrogen and P=O group to silicon activates HSiCl₃, enabling selective aldehyde reduction .
-
Efficiency :
-
Comparative Performance :
Table 2: Catalyst Performance in Hydrosilylation
Catalyst | Conversion (%) | Selectivity (Aldehyde/Ketone) |
---|---|---|
This compound | 80 | 100/0 |
Diethyl 3-pyridylphosphonate | 47 | 100/0 |
Diethyl 4-pyridylphosphonate | 33 | 100/0 |
Diethyl 2-pyridylthiophosphonate | 8 | 100/0 |
Reaction Optimization and Limitations
-
Temperature Sensitivity : Reactions typically proceed at 60–110°C, with higher temperatures accelerating decomposition in some substrates .
-
Solvent Dependence : Polar aprotic solvents (e.g., DMF, dichloromethane) enhance reaction rates and yields .
-
Substrate Limitations : Bulky substrates (e.g., 2,6-dibromopyridine) show reduced yields due to steric hindrance .
This compound’s dual functionality enables its use in cross-coupling and catalytic applications, with structural analogs providing insights into reactivity trends. Experimental data underscore its efficiency and selectivity, positioning it as a valuable reagent in synthetic chemistry.
Scientific Research Applications
Medicinal Chemistry
Diethyl 2-pyridylphosphonate has shown promise in medicinal chemistry, particularly in the development of pharmaceuticals. Its structural similarity to biologically active phosphonates allows it to interact with various biological targets, including enzymes involved in metabolic pathways. This interaction suggests potential uses as enzyme inhibitors and in drug development.
Case Study: Drug Resistance Alleviation
Recent studies have indicated that derivatives of pyridyl phosphonates, including this compound, can alleviate drug resistance in cancer treatments. For instance, the compound PAK-104P, a pyridyl phosphonate, has been shown to enhance the efficacy of drugs like paclitaxel and doxorubicin by overcoming resistance mechanisms .
Catalysis
The compound serves as a catalyst in several reactions, particularly in hydrosilylation processes where it enhances both efficiency and selectivity. Its catalytic properties are attributed to the presence of the phosphorus atom, which can stabilize transition states during chemical reactions.
Table 1: Catalytic Applications of this compound
Reaction Type | Role of this compound | Outcome |
---|---|---|
Hydrosilylation | Catalyst | Improved efficiency and selectivity |
Enzyme inhibition | Inhibitor | Potential therapeutic applications |
Synthesis of phosphonates | Reactant | High yields in various synthetic pathways |
Agricultural Applications
The compound has potential applications as a herbicide due to its ability to inhibit specific enzymes that are crucial for plant growth. Research into its efficacy against various weed species is ongoing, highlighting its role in developing environmentally friendly agricultural practices.
Material Science
This compound is also utilized in material science for the synthesis of phosphorus-containing polymers and dendrimers. These materials exhibit unique properties that can be tailored for specific applications, such as drug delivery systems or advanced coatings .
Case Study: Polymer Synthesis
Research has demonstrated that incorporating this compound into polymer matrices can enhance their mechanical properties and thermal stability, making them suitable for high-performance applications .
Mechanism of Action
The mechanism of action of diethyl 2-pyridylphosphonate involves its role as a bidentate Lewis base catalyst. It activates hydrosilanes by coordinating to the silicon atom, thereby facilitating the transfer of the hydride to the substrate. This activation is crucial for the hydrosilylation of aldehydes and other related reactions .
Comparison with Similar Compounds
The catalytic performance and chemical behavior of Diethyl 2-pyridylphosphonate are strongly influenced by its pyridyl-phosphonate structure. Below is a systematic comparison with structurally related compounds:
Table 1: Comparative Analysis of this compound and Analogous Compounds
Key Findings:
Positional Isomerism (2- vs. 3-/4-Pyridyl):
The 2-pyridyl group in this compound enables bidentate coordination to HSiCl₃, enhancing its Lewis basicity and catalytic efficiency. In contrast, 3- and 4-pyridyl isomers lack optimal geometry for dual coordination, resulting in reduced activity .
Donor Atom Effects (Phosphonate vs. Thiophosphonate): Replacing the phosphonate oxygen with sulfur (as in Diethyl 2-pyridylthiophosphonate) diminishes electron-donating capacity, leading to weaker activation of HSiCl₃ and lower catalytic performance .
Aromatic vs. Aliphatic Substituents: Non-pyridyl analogs like Diethyl phenylphosphonate or Diethyl allylphosphonate lack the pyridine ring’s nitrogen lone pair, which is critical for substrate activation. These compounds exhibit negligible catalytic activity in hydrosilylation .
Biological vs. Catalytic Applications:
Compounds such as Diethyl (2-methylpyrrolidin-2-yl)phosphate are tailored for biological roles (e.g., reperfusion injury markers) due to their pyrrolidine ring, which enhances solubility and interaction with cellular targets .
Research Findings and Mechanistic Insights
Mechanistic Superiority of this compound
The 2-pyridyl group acts as a dual Lewis base, coordinating to HSiCl₃ to form a hypervalent silicon intermediate. This activation lowers the transition-state energy for hydride transfer to aldehydes, achieving high chemoselectivity (e.g., reduction of benzaldehyde over acetophenone) .
Steric and Electronic Modifications in Analogs
- Electron-Withdrawing Groups (e.g., Bromo): Derivatives like Diethyl ((6-bromopyridin-2-yl)fluoromethyl)phosphonate () may exhibit reduced catalytic activity due to decreased electron density on the pyridine nitrogen, though direct studies are lacking.
Q & A
Basic Research Questions
Q. What are the established synthetic routes for Diethyl 2-pyridylphosphonate, and how do they compare in efficiency?
this compound is traditionally synthesized via nucleophilic substitution between 2-picolyl chloride and triethylphosphite. However, this method requires unstable and costly starting materials . An alternative one-pot reaction using α-picoline and diethyl chlorophosphate has been developed, offering improved stability and accessibility. The latter method avoids isolation of intermediates, streamlining synthesis under mild conditions (room temperature, no stringent inert atmosphere required) . Researchers should compare yields and purity using techniques like P NMR to assess phosphonate formation efficiency.
Q. How can spectroscopic methods characterize this compound and its derivatives?
- H NMR : Pyridyl protons resonate between δ 7.5–8.5 ppm, while phosphonate-linked methyl/methylene groups appear as multiplets near δ 4.0–4.2 ppm. For example, a derivative (Diethyl 3,4-dimethyl-2-pyridinylphosphonate) shows pyridyl protons at δ 8.01 (d, J = 9.0 Hz) and phosphonate ethyl groups at δ 1.24 (t, J = 6.8 Hz) .
- Mass Spectrometry : ESI–MS often detects [M+H] ions (e.g., m/z 287 for a derivative with molecular weight 286) .
- Infrared Spectroscopy : P=O stretches typically occur at 1250–1200 cm, while pyridyl C-N/C=C vibrations appear at 1600–1450 cm .
Q. What safety protocols are critical when handling this compound?
While specific safety data for this compound is limited, general precautions for phosphonates include:
- PPE : Nitrile gloves, lab coats, and safety goggles to prevent skin/eye contact .
- Ventilation : Use fume hoods to avoid inhalation of vapors/aerosols .
- Spill Management : Absorb with inert material (e.g., sand) and dispose as hazardous waste .
Advanced Research Questions
Q. How can researchers resolve contradictions in spectroscopic data for this compound derivatives?
Conflicting NMR or IR results often arise from solvent effects, tautomerism, or impurities. For example:
- Variable Solvent Effects : Deuterated chloroform (CDCl) vs. DMSO-d can shift pyridyl proton signals by 0.1–0.3 ppm. Validate assignments using 2D NMR (e.g., H-C HSQC) .
- Tautomeric Equilibria : Pyridyl-phosphonate derivatives may exhibit keto-enol tautomerism, altering P NMR chemical shifts. Monitor pH and temperature to stabilize specific forms .
Q. What experimental strategies optimize this compound’s reactivity in Wittig-Horner reactions?
Phase-transfer catalysis (PTC) enhances reactivity in biphasic systems. For example:
- Catalyst Selection : Use tetrabutylammonium bromide (TBAB) to shuttle deprotonated phosphonate anions into organic phases .
- Base Optimization : LiN(iPr) (LDA) at –78°C improves regioselectivity in alkyne additions, minimizing side reactions .
- Workflow : Quench reactions with 1 M HCl to protonate intermediates, followed by extraction with non-polar solvents (e.g., petroleum ether) .
Q. How can computational modeling predict the reactivity of this compound in cross-coupling reactions?
- DFT Calculations : Analyze frontier molecular orbitals (HOMO/LUMO) to predict nucleophilic/electrophilic sites. The pyridyl nitrogen’s lone pair (HOMO) often directs electrophilic attacks .
- Topological Polar Surface Area (TPSA) : A TPSA of ~50 Ų (calculated for derivatives) correlates with membrane permeability, aiding drug-design applications .
- Molecular Dynamics : Simulate solvent interactions to optimize reaction media (e.g., THF vs. DMF) for coupling efficiency .
Q. Data Contradiction Analysis
Q. Why might 31^{31}31P NMR spectra show unexpected peaks during synthesis?
Common artifacts include:
- Phosphonate Hydrolysis : Trace moisture hydrolyzes P-OEt groups to phosphoric acid derivatives (δ 0–10 ppm). Use anhydrous solvents and molecular sieves .
- Byproduct Formation : Unreacted triethylphosphite (δ 128–130 ppm) or oxidized species (δ 18–22 ppm) indicate incomplete purification. Reflux times or column chromatography (silica gel, ethyl acetate/hexane) may resolve this .
Q. Methodological Recommendations
- Synthesis Optimization : Replace traditional 2-picolyl chloride with α-picoline and diethyl chlorophosphate for cost-effective, scalable synthesis .
- Analytical Cross-Validation : Combine H NMR, P NMR, and IR to confirm structural integrity and purity .
- Safety Compliance : Adopt protocols from analogous phosphonates (e.g., diethyl ketomalonate) for handling and disposal .
Properties
Molecular Formula |
C9H14NO3P |
---|---|
Molecular Weight |
215.19 g/mol |
IUPAC Name |
2-diethoxyphosphorylpyridine |
InChI |
InChI=1S/C9H14NO3P/c1-3-12-14(11,13-4-2)9-7-5-6-8-10-9/h5-8H,3-4H2,1-2H3 |
InChI Key |
ANRDLKWBUFTLIB-UHFFFAOYSA-N |
Canonical SMILES |
CCOP(=O)(C1=CC=CC=N1)OCC |
Origin of Product |
United States |
Synthesis routes and methods
Procedure details
Disclaimer and Information on In-Vitro Research Products
Please be aware that all articles and product information presented on BenchChem are intended solely for informational purposes. The products available for purchase on BenchChem are specifically designed for in-vitro studies, which are conducted outside of living organisms. In-vitro studies, derived from the Latin term "in glass," involve experiments performed in controlled laboratory settings using cells or tissues. It is important to note that these products are not categorized as medicines or drugs, and they have not received approval from the FDA for the prevention, treatment, or cure of any medical condition, ailment, or disease. We must emphasize that any form of bodily introduction of these products into humans or animals is strictly prohibited by law. It is essential to adhere to these guidelines to ensure compliance with legal and ethical standards in research and experimentation.