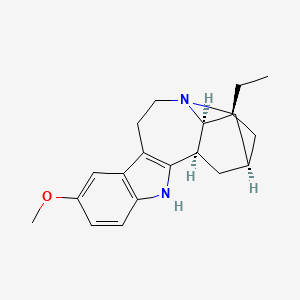
10-Methoxyibogamine
Overview
Description
10-Methoxyibogamine, commonly known as ibogaine (C₂₀H₂₆N₂O), is a naturally occurring monoterpenoid indole alkaloid found in plants of the Apocynaceae family, including Tabernanthe iboga, Voacanga africana, and Tabernaemontana species . It is renowned for its multifaceted pharmacological properties:
- Psychedelic and dissociative effects: At high doses, it induces prolonged hallucinogenic states (18–36 hours) .
- Cytotoxic activity: Exhibits toxicity against cancer cell lines like A2780 ovarian cancer (IC₅₀: 3.5–11 mg/mL) .
Its synthesis was first described in 1957, and its structure was confirmed via X-ray crystallography in 1958 . Despite therapeutic promise, ibogaine’s cardiac toxicity and hallucinogenic side effects limit clinical use, driving research into analogs with improved safety .
Preparation Methods
Ibogaine can be obtained through extraction from plants in the Apocynaceae family, such as Tabernanthe iboga, Voacanga africana, and Tabernaemontana undulata . The total synthesis of ibogaine was first described in 1956 . The synthetic route involves several steps, including the use of voacangine as a precursor . Industrial production methods often involve semi-synthesis from voacangine due to the complexity of the total synthesis .
Chemical Reactions Analysis
Enzymatic Catalysis in Final Steps
The final biosynthetic steps are catalyzed by I10H (ibogamine-10-hydroxylase) and N10OMT (noribogaine-10-O-methyltransferase):
3.1 Substrate Specificity
-
I10H :
-
N10OMT :
3.2 Reaction Kinetics
Enzyme | Substrate | Kₘ (μM) | Vₘₐₓ (pmol s⁻¹ mg⁻¹) | Catalytic Efficiency (m⁻¹ s⁻¹) |
---|---|---|---|---|
I10H | Ibogamine | 11.4 ± 1.87 | N/A (microsomal) | N/A |
N10OMT | Noribogaine | 73.6 ± 28.5 | 14 ± 1.84 | 7.45 ± 3.05 |
3.3 pH Optima
Metabolic Pathway
In humans, ibogaine is metabolized primarily by CYP2D6 to its active metabolite noribogaine (10-hydroxyibogaine) .
Step | Enzyme | Product |
---|---|---|
Ibogaine → Noribogaine | CYP2D6 | Psychoactive metabolite |
Noribogaine exhibits prolonged activity due to its longer half-life .
Pharmacological Interactions
Ibogaine and noribogaine bind to multiple receptors with varying affinities:
Site | Ibogaine Kᵢ (nM) | Noribogaine Kᵢ (nM) |
---|---|---|
μ-Opioid (MOR) | 2,000–100,000 | 700–3,000 |
κ-Opioid (KOR) | 2,000–4,000 | 600–1,000 |
NMDA | 1,000–3,000 | 6,000–15,000 |
SERT | 500 | 40 |
This multi-target interaction underpins its psychoactive and anti-addictive effects .
Key Research Findings
-
Enzymatic Dual Role : I10H and N10OMT catalyze both branches of ibogaine biosynthesis, enabling parallel pathways via voacangine or ibogamine .
-
Regiospecificity : The enzymes exclusively target the 10-position of the ibogan scaffold, ensuring precise methylation/hydroxylation .
-
Metabolic Stability : Noribogaine persists in the body for days, contributing to ibogaine’s prolonged pharmacological effects .
This comprehensive analysis highlights the intricate chemical and enzymatic processes governing 10-methoxyibogamine’s synthesis, metabolism, and biological activity.
Scientific Research Applications
Chemical Properties and Biosynthesis
10-Methoxyibogamine is synthesized through the metabolic processes involving enzymes such as ibogamine-10-hydroxylase and noribogaine-10-O-methyltransferase. These enzymes are crucial in the biosynthesis of ibogaine from Tabernanthe iboga, where they facilitate the final hydroxylation and methylation steps, leading to the production of various alkaloids including this compound .
Anti-Addictive Properties
Research indicates that ibogaine and its derivatives, including this compound, exhibit significant anti-addictive properties. A study highlighted that participants undergoing ibogaine treatment reported substantial reductions in opioid withdrawal symptoms and cravings. Specifically, 80% of participants noted a drastic reduction in withdrawal symptoms, with 30% achieving long-term abstinence from opioids .
Table 1: Summary of Findings on Ibogaine Treatment for Addiction
Study Reference | Participant Profile | Key Findings |
---|---|---|
Glue et al. (2015) | Opioid users (n=88) | 72% had used opioids for >4 years; 80% reported reduced withdrawal symptoms. |
Bastiaans (2004) | Mixed substance users | 24% achieved complete abstinence; 33% reduced usage. |
PMC6157925 | Opioid users | 54% remained abstinent for at least one year post-treatment. |
Neuropharmacological Mechanisms
The pharmacodynamics of this compound suggest it interacts with multiple neurotransmitter systems. It has shown to act as a serotonin reuptake inhibitor and has moderate affinity for kappa-opioid receptors, which may contribute to its psychoactive effects . The compound's ability to modulate these pathways is crucial for its therapeutic efficacy in treating substance use disorders.
Case Study: Long-Term Effects of Ibogaine Treatment
A longitudinal study assessed the long-term outcomes of ibogaine treatment among opioid users. Participants reported improvements not only in substance use but also in psychological well-being, with significant reductions in anxiety and depression scores post-treatment . This underscores the potential for this compound as a therapeutic agent in managing both addiction and associated mental health conditions.
Clinical Observations
In clinical settings, patients receiving ibogaine treatment often report profound psychological experiences that contribute to their recovery process. Anecdotal evidence suggests that the spiritual significance attributed to the treatment plays a role in its effectiveness, highlighting the need for further research into the subjective experiences associated with this compound treatment .
Future Directions and Research Opportunities
The exploration of this compound's applications is still in its infancy. Future research could focus on:
- Mechanistic Studies : Understanding the specific pathways through which this compound exerts its effects.
- Comparative Studies : Evaluating its efficacy against other anti-addictive treatments.
- Safety Profiles : Longitudinal studies assessing the safety and side effects associated with chronic use.
Mechanism of Action
Ibogaine exerts its effects by interacting with various neurotransmitter systems, including serotonin, dopamine, and glutamate receptors . It acts as a noncompetitive antagonist at the nicotinic acetylcholine receptors, specifically the α3β4 subtype, which is implicated in mitigating opioid withdrawal symptoms . Ibogaine is rapidly metabolized into noribogaine, which also contributes to its pharmacological effects .
Comparison with Similar Compounds
Comparison with Structurally Similar Compounds
Ibogamine
- Structure : Lacks the 10-methoxy group present in 10-methoxyibogamine.
- Source : Naturally occurs in Tabernaemontana species .
- Pharmacology: Weaker cholinesterase inhibition compared to this compound . Limited psychedelic activity, suggesting the 10-methoxy group is critical for hallucinogenic effects .
- Cytotoxicity : Similar potency against A2780 ovarian cancer cells (IC₅₀: ~5 mg/mL) .
11-Methoxyibogamine
- Structure : Methoxy group at position 11 instead of 10.
- Source : Isolated from Tabernaemontana elegans and T. calcarea .
- Pharmacology :
12-Methoxyibogamine
- Structure: Methoxy group at position 12 (Note: Structural ambiguity exists; some sources may conflate numbering systems) .
- Synthesis : Produced via a 13-step racemic synthesis starting from nicotinamide .
- Pharmacology: Limited data, but preliminary studies suggest weaker central nervous system activity than this compound .
Comparison with Functionally Related Alkaloids
Coronaridine
- Structure : Shares the ibogamine core but lacks methoxy groups.
- Source : Found in Tabernaemontana catharinensis .
- Pharmacology: Antitumor activity: Cytotoxic against Hep-2 laryngeal carcinoma (IC₅₀: 8.2 µg/mL) .
Voacangine
- Structure : Contains a methoxy group at position 11 and an additional hydroxyl group.
- Source : Abundant in Voacanga africana .
- Pharmacology :
18-Methoxycoronaridine (18-MC)
- Structure : Synthetic analog with a methoxy group at position 18 and a methyl ester .
- Synthesis : Developed to retain anti-addictive properties while minimizing toxicity .
- Pharmacology: Safety: No reported cardiotoxicity or hallucinations in preclinical studies . Efficacy: Equipotent to this compound in reducing cocaine self-administration in rodents .
Data Tables
Table 1: Structural and Pharmacological Comparison
Q & A
Basic Research Questions
Q. What are the established methodologies for synthesizing and characterizing 10-Methoxyibogamine in academic research?
Synthesis of this compound typically involves alkaloid isolation from natural sources (e.g., Tabernanthe iboga) or semi-synthetic modification of ibogaine. For novel compounds, structural characterization requires nuclear magnetic resonance (NMR), high-resolution mass spectrometry (HRMS), and chromatographic purity analysis (HPLC/GC-MS). Researchers must provide full spectral data for new derivatives, including -NMR, -NMR, and IR spectra, alongside elemental analysis to confirm purity ≥95% . Known compounds require citation of prior synthesis protocols to avoid redundancy .
Q. How should researchers design experiments to assess the pharmacological profile of this compound?
Pharmacological studies should include in vitro assays (e.g., receptor binding affinity at serotonin, NMDA, or opioid receptors) and in vivo behavioral models (e.g., conditioned place preference for addiction studies). Dose-response curves, IC/EC values, and comparison to positive/negative controls (e.g., ibogaine for mechanistic contrast) are critical. Ensure statistical rigor by predefining sample sizes, randomization protocols, and blinding procedures to minimize bias .
Q. What ethical considerations apply to preclinical studies involving this compound?
Animal studies must comply with institutional review board (IRB) guidelines, emphasizing humane endpoints, minimization of distress, and justification of species selection. For human cell lines, obtain ethical approval for sourcing and usage. Declare conflicts of interest and adhere to ARRIVE guidelines for reporting .
Advanced Research Questions
Q. How can researchers resolve contradictions in reported mechanisms of action for this compound?
Contradictory findings (e.g., serotonergic vs. glutamatergic modulation) may arise from differences in experimental models (cell lines vs. whole organisms) or dosing regimens. Conduct systematic reviews using frameworks like PRISMA to synthesize evidence, followed by hypothesis-driven experiments (e.g., receptor knockout models or selective antagonist co-administration) to isolate pathways .
Q. What strategies optimize the reproducibility of this compound’s anti-addictive effects in translational research?
Reproducibility requires detailed reporting of experimental conditions (e.g., solvent used, administration route, animal strain). Share raw data and protocols via repositories like Zenodo. Use the FINER criteria (Feasible, Interesting, Novel, Ethical, Relevant) to validate translational relevance and align with clinical trial design principles .
Q. How can computational modeling enhance the understanding of this compound’s pharmacokinetics?
Molecular dynamics simulations and QSAR (Quantitative Structure-Activity Relationship) models predict metabolic stability, blood-brain barrier permeability, and metabolite formation. Validate predictions with in vitro microsomal assays (e.g., CYP450 enzyme inhibition) and in vivo pharmacokinetic profiling (AUC, , bioavailability) .
Q. Methodological Challenges
Q. What are best practices for synthesizing literature on this compound’s neuropharmacological effects?
Conduct scoping reviews to map evidence gaps, followed by meta-analyses for quantitative synthesis. Use PICO (Population, Intervention, Comparison, Outcome) to frame research questions, e.g., “Does this compound (Intervention) reduce opioid withdrawal symptoms (Outcome) in rodent models (Population) compared to ibogaine (Comparison)?” .
Q. How should researchers address variability in compound purity across studies?
Standardize purity verification using orthogonal methods (HPLC + NMR) and report batch-specific data. For natural extracts, quantify this compound via LC-MS and disclose co-occurring alkaloids (e.g., ibogaine, noribogaine) that may confound results .
Q. What statistical approaches are recommended for analyzing dose-dependent behavioral outcomes?
Non-linear regression models (e.g., log-dose vs. response) and ANOVA with post-hoc corrections (e.g., Tukey’s test) are standard. For longitudinal data, mixed-effects models account for intra-subject variability. Pre-register analysis plans to mitigate p-hacking .
Q. Emerging Research Directions
Q. How can CRISPR-Cas9 models elucidate this compound’s molecular targets?
Generate knockout cell lines for candidate receptors (e.g., 5-HT, κ-opioid) and assess compound efficacy via calcium imaging or cAMP assays. Combine with transcriptomics (RNA-seq) to identify downstream pathways .
Q. What in silico tools predict this compound’s potential off-target effects?
Use SwissTargetPrediction or SEA (Similarity Ensemble Approach) to forecast off-target binding. Validate with high-throughput screening panels (e.g., Eurofins SafetyScreen44) .
Properties
Key on ui mechanism of action |
... In this study, /researchers/ first characterized the actions of ibogaine on ethanol self-administration in rodents. Ibogaine decreased ethanol intake by rats in two-bottle choice and operant self-administration paradigms. Ibogaine also reduced operant self-administration of ethanol in a relapse model. Next, /the researchers/ identified a molecular mechanism that mediates the desirable activities of ibogaine on ethanol intake. Microinjection of ibogaine into the ventral tegmental area (VTA), but not the substantia nigra, reduced self-administration of ethanol, and systemic administration of ibogaine increased the expression of glial cell line-derived neurotrophic factor (GDNF) in a midbrain region that includes the VTA. In dopaminergic neuron-like SHSY5Y cells, ibogaine treatment upregulated the GDNF pathway as indicated by increases in phosphorylation of the GDNF receptor, Ret, and the downstream kinase, ERK1 (extracellular signal-regulated kinase 1). Finally, the ibogaine-mediated decrease in ethanol self-administration was mimicked by intra-VTA microinjection of GDNF and was reduced by intra-VTA delivery of anti-GDNF neutralizing antibodies. Together, these results suggest that GDNF in the VTA mediates the action of ibogaine on ethanol consumption. These findings highlight the importance of GDNF as a new target for drug development for alcoholism that may mimic the effect of ibogaine against alcohol consumption but avoid the negative side effects. Ibogaine (Endabuse) is a psychoactive indole alkaloid found in the West African shrub, Tabernanthe iboga. This drug interrupts cocaine and amphetamine abuse and has been proposed for treatment of addiction to these stimulants. However, the mechanism of action that explains its pharmacological properties is unclear. Since previous studies demonstrated differential effects of psychotomimetic drugs (cocaine and methamphetamine) on neuropeptides such as neurotensin (NT), the present study was designed to determine: (1) the effects of ibogaine on striatal, nigral, cortical, and accumbens neurotensin-like immunoreactivity (NTLI); (2) the effects of selective dopamine antagonists on ibogaine-induced changes in NT concentrations in these brain areas; and (3) the effects of ibogaine pretreatment on cocaine-induced changes in striatal, nigral, cortical and accumbens NTLI content. Ibogaine treatments profoundly affected NT systems by increasing striatal, nigral, and accumbens NTLI content 12 h after the last drug administration. In contrast, NTLI concentrations were not significantly increased in the frontal cortex after ibogaine treatment. The ibogaine-induced increases in NTLI in striatum, nucleus accumbens and substantia nigra were blocked by coadministration of the selective D1 receptor antagonist, SCH 23390. The D2 receptor antagonist, eticlopride, blocked the ibogaine-induced increase in nigral NTLI, but not in striatum and nucleus accumbens. Ibogaine pretreatment significantly blocked the striatal and nigral increases of NTLI resulting from a single cocaine administration. Whereas many of the responses by NT systems to ibogaine resembled those which occur after cocaine, there were also some important differences. These data suggest that NT may contribute to an interaction between ibogaine and the DA system and may participate in the pharmacological actions of this drug. Ibogaine, a putative antiaddictive drug, is remarkable in its apparent ability to downgrade withdrawal symptoms and drug craving for extended periods of time after a single dose. Ibogaine acts as a non-competitive NMDA receptor antagonist, while NMDA has been implicated in long lasting changes in neuronal function and in the physiological basis of drug addiction. The purpose of this study was to verify if persistent changes in NMDA receptors could be shown in vivo and in vitro after a single administration of ibogaine. The time course of ibogaine effects were examined on NMDA-induced seizures and (3)H MK-801 binding to cortical membranes in mice 30 min, 24, 48, and 72 hr post treatment. Ibogaine (80 mg/kg, ip) was effective in inhibiting convulsions induced by NMDA at 24 and 72 hours post administration. Likewise, (3)H MK-801 binding was significantly decreased at 24 and 72 hr post ibogaine. No significant differences from controls were found at 30 min or 48 h post ibogaine. This long lasting and complex pattern of modulation of NMDA receptors prompted by a single dose of ibogaine may be associated to its antiaddictive properties. The iboga alkaloids are a class of small molecules defined structurally on the basis of a common ibogamine skeleton, some of which modify opioid withdrawal and drug self-administration in humans and preclinical models. These compounds may represent an innovative approach to neurobiological investigation and development of addiction pharmacotherapy. In particular, the use of the prototypic iboga alkaloid ibogaine for opioid detoxification in humans raises the question of whether its effect is mediated by an opioid agonist action, or if it represents alternative and possibly novel mechanism of action. The aim of this study was to independently replicate and extend evidence regarding the activation of mu-opioid receptor (MOR)-related G proteins by iboga alkaloids. Ibogaine, its major metabolite noribogaine, and 18-methoxycoronaridine (18-MC), a synthetic congener, were evaluated by agonist-stimulated guanosine-5'-O-(gamma-thio)-triphosphate (((35)S)GTPyS) binding in cells overexpressing the recombinant MOR, in rat thalamic membranes, and autoradiography in rat brain slices. In rat thalamic membranes ibogaine, noribogaine and 18-MC were MOR antagonists with functional Ke values ranging from 3 uM (ibogaine) to 13 uM (noribogaine and 18MC). Noribogaine and 18-MC did not stimulate ((35)S)GTPyS binding in Chinese hamster ovary cells expressing human or rat MORs, and had only limited partial agonist effects in human embryonic kidney cells expressing mouse MORs. Ibogaine did not did not stimulate ((35)S)GTPyS binding in any MOR expressing cells. Noribogaine did not stimulate ((35)S)GTPyS binding in brain slices using autoradiography. An MOR agonist action does not appear to account for the effect of these iboga alkaloids on opioid withdrawal. Taken together with existing evidence that their mechanism of action also differs from that of other non-opioids with clinical effects on opioid tolerance and withdrawal, these findings suggest a novel mechanism of action, and further justify the search for alternative targets of iboga alkaloids. For more Mechanism of Action (Complete) data for Ibogaine (12 total), please visit the HSDB record page. |
---|---|
Molecular Formula |
C20H26N2O |
Molecular Weight |
310.4 g/mol |
IUPAC Name |
(1R,15S,17S,18S)-17-ethyl-7-methoxy-3,13-diazapentacyclo[13.3.1.02,10.04,9.013,18]nonadeca-2(10),4(9),5,7-tetraene |
InChI |
InChI=1S/C20H26N2O/c1-3-13-8-12-9-17-19-15(6-7-22(11-12)20(13)17)16-10-14(23-2)4-5-18(16)21-19/h4-5,10,12-13,17,20-21H,3,6-9,11H2,1-2H3/t12-,13-,17-,20-/m0/s1 |
InChI Key |
HSIBGVUMFOSJPD-NXWOVTFFSA-N |
SMILES |
CCC1CC2CC3C1N(C2)CCC4=C3NC5=C4C=C(C=C5)OC |
Isomeric SMILES |
CC[C@H]1C[C@H]2C[C@@H]3[C@H]1N(C2)CCC4=C3NC5=C4C=C(C=C5)OC |
Canonical SMILES |
CCC1CC2CC3C1N(C2)CCC4=C3NC5=C4C=C(C=C5)OC |
melting_point |
148 °C Crystals from ethyl acetate. mp: 132 °C /Ibopamine hydrochloride/ |
solubility |
Soluble in chloroform |
Origin of Product |
United States |
Retrosynthesis Analysis
AI-Powered Synthesis Planning: Our tool employs the Template_relevance Pistachio, Template_relevance Bkms_metabolic, Template_relevance Pistachio_ringbreaker, Template_relevance Reaxys, Template_relevance Reaxys_biocatalysis model, leveraging a vast database of chemical reactions to predict feasible synthetic routes.
One-Step Synthesis Focus: Specifically designed for one-step synthesis, it provides concise and direct routes for your target compounds, streamlining the synthesis process.
Accurate Predictions: Utilizing the extensive PISTACHIO, BKMS_METABOLIC, PISTACHIO_RINGBREAKER, REAXYS, REAXYS_BIOCATALYSIS database, our tool offers high-accuracy predictions, reflecting the latest in chemical research and data.
Strategy Settings
Precursor scoring | Relevance Heuristic |
---|---|
Min. plausibility | 0.01 |
Model | Template_relevance |
Template Set | Pistachio/Bkms_metabolic/Pistachio_ringbreaker/Reaxys/Reaxys_biocatalysis |
Top-N result to add to graph | 6 |
Feasible Synthetic Routes
Disclaimer and Information on In-Vitro Research Products
Please be aware that all articles and product information presented on BenchChem are intended solely for informational purposes. The products available for purchase on BenchChem are specifically designed for in-vitro studies, which are conducted outside of living organisms. In-vitro studies, derived from the Latin term "in glass," involve experiments performed in controlled laboratory settings using cells or tissues. It is important to note that these products are not categorized as medicines or drugs, and they have not received approval from the FDA for the prevention, treatment, or cure of any medical condition, ailment, or disease. We must emphasize that any form of bodily introduction of these products into humans or animals is strictly prohibited by law. It is essential to adhere to these guidelines to ensure compliance with legal and ethical standards in research and experimentation.