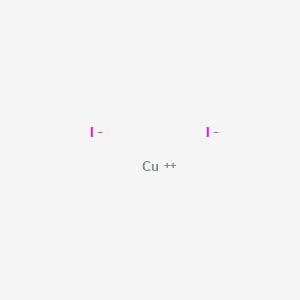
Copper iodide
- Click on QUICK INQUIRY to receive a quote from our team of experts.
- With the quality product at a COMPETITIVE price, you can focus more on your research.
Overview
Description
Copper iodide, also known as cuprous iodide, is an inorganic compound with the chemical formula CuI. It is a white or slightly yellowish solid at room temperature. When exposed to light, it slowly turns brown due to the liberation of iodine. This compound is not readily soluble in water but can dissolve in solutions containing iodide ions or in concentrated halide solutions. This compound is used in various applications, ranging from organic synthesis to cloud seeding .
Preparation Methods
Copper iodide can be prepared through several synthetic routes. In the laboratory, it is commonly prepared by mixing an aqueous solution of potassium iodide with a soluble copper(II) salt such as copper(II) sulfate. The reaction can be represented as: [ \text{CuSO}_4 + 2 \text{KI} \rightarrow \text{CuI}_2 + \text{K}_2\text{SO}_4 ]
Another method involves the direct reaction of iodine with copper: [ \text{Cu} + \text{I}_2 \rightarrow \text{CuI} ]
Industrial production often involves the reaction of copper sulfate with potassium iodide, where this compound precipitates out of the solution and can then be collected .
Chemical Reactions Analysis
Copper iodide undergoes various chemical reactions, including oxidation, reduction, and substitution reactions. Some common reactions include:
-
Oxidation: : this compound can be oxidized to copper(II) iodide and iodine. [ 2 \text{CuI} \rightarrow \text{Cu}_2\text{I}_2 + \text{I}_2 ]
-
Reduction: : this compound can be reduced to metallic copper. [ \text{CuI} + \text{H}_2 \rightarrow \text{Cu} + \text{HI} ]
-
Substitution: : this compound can react with various organic and inorganic reagents to form different products. For example, it can react with aryl iodides to form aryl sulfides. [ \text{CuI} + \text{ArI} \rightarrow \text{ArCuI} ]
Common reagents used in these reactions include iodine, hydrogen, and aryl iodides. The major products formed from these reactions include copper(II) iodide, iodine, metallic copper, and aryl sulfides .
Scientific Research Applications
Copper iodide has a wide range of scientific research applications:
-
Chemistry: : It is used as a catalyst in organic synthesis, including coupling reactions such as Suzuki, Stille, Sonogashira, Heck, and Ullmann reactions. It is also used in living radical polymerizations and cross-coupling reactions .
-
Biology and Medicine: : this compound serves as a source of iodine in antiseptic solutions. Its ability to slowly release iodine makes it ideal for sustained topical applications .
-
Industry: : this compound is used in the fabrication of solar cells, photoluminescent materials, and nanocrystal synthesis. It is also used in cloud seeding, where iodide ions act as nuclei around which water vapor can condense, promoting rainfall .
Mechanism of Action
The mechanism of action of copper iodide involves its ability to participate in various chemical reactions. In organic synthesis, it acts as a catalyst by facilitating the formation of bonds between different molecules. In biological applications, this compound releases iodine, which has antiseptic properties. The molecular targets and pathways involved include the formation of copper-iodine bonds and the release of iodine ions .
Comparison with Similar Compounds
Copper iodide can be compared with other similar compounds such as copper(I) fluoride, copper(I) chloride, and copper(I) bromide. These compounds share similar properties, such as being inorganic polymers and having a rich phase diagram with several crystalline forms. copper iod
Properties
Molecular Formula |
CuI2 |
---|---|
Molecular Weight |
317.35 g/mol |
IUPAC Name |
copper;diiodide |
InChI |
InChI=1S/Cu.2HI/h;2*1H/q+2;;/p-2 |
InChI Key |
GBRBMTNGQBKBQE-UHFFFAOYSA-L |
Canonical SMILES |
[Cu+2].[I-].[I-] |
Origin of Product |
United States |
Disclaimer and Information on In-Vitro Research Products
Please be aware that all articles and product information presented on BenchChem are intended solely for informational purposes. The products available for purchase on BenchChem are specifically designed for in-vitro studies, which are conducted outside of living organisms. In-vitro studies, derived from the Latin term "in glass," involve experiments performed in controlled laboratory settings using cells or tissues. It is important to note that these products are not categorized as medicines or drugs, and they have not received approval from the FDA for the prevention, treatment, or cure of any medical condition, ailment, or disease. We must emphasize that any form of bodily introduction of these products into humans or animals is strictly prohibited by law. It is essential to adhere to these guidelines to ensure compliance with legal and ethical standards in research and experimentation.