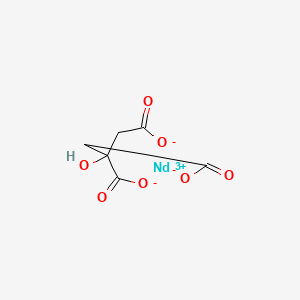
Neodymium citrate
- Click on QUICK INQUIRY to receive a quote from our team of experts.
- With the quality product at a COMPETITIVE price, you can focus more on your research.
Overview
Description
Neodymium citrate is a useful research compound. Its molecular formula is C6H5NdO7 and its molecular weight is 333.34 g/mol. The purity is usually 95%.
BenchChem offers high-quality this compound suitable for many research applications. Different packaging options are available to accommodate customers' requirements. Please inquire for more information about this compound including the price, delivery time, and more detailed information at info@benchchem.com.
Scientific Research Applications
Materials Science
1.1 Supercapacitors and Energy Storage
Recent research highlights the synthesis of neodymium gallium oxides via a citrate-gel-matrix method, which shows promising applications in supercapacitors. These materials exhibit high specific capacitance and electrochemical stability, making them suitable for energy storage devices. The tunability of their morphology and composition allows for enhanced performance in asymmetric supercapacitor applications .
1.2 Biodegradable Implant Materials
Neodymium citrate is being investigated as a component in biodegradable implant materials. Its low toxicity and favorable mechanical properties make it a candidate for temporary implants that support bone healing while gradually degrading without adverse effects . Studies on magnesium alloys incorporating neodymium have shown promising results regarding their mechanical integrity and biocompatibility during degradation processes .
Biochemistry
2.1 Complexation Studies
This compound forms stable complexes with various ligands, including HEDTA (N-(2-hydroxyethyl)ethylenediaminetriacetic acid). Spectrophotometric titration experiments have identified a ternary neodymium-HEDTA-citrate complex, revealing insights into the stoichiometry and stability of these complexes. The formation of such complexes is significant for understanding the bioavailability of neodymium in biological systems .
2.2 Contrast Agents in Electron Microscopy
This compound is being explored as a non-toxic alternative to traditional uranium-based contrast agents in electron microscopy. Its low toxicity and effective contrast capabilities allow for enhanced imaging without the associated risks of radioactive materials . This application is particularly valuable in biological research where safety is paramount.
Environmental Applications
3.1 Recovery of Rare Earth Elements
This compound plays a role in the leaching and recovery processes of rare earth elements from industrial waste, such as NdFeB magnets. Research indicates that citric acid can effectively extract neodymium from these materials, providing an environmentally friendly method for recycling valuable resources . The slow leaching kinetics observed necessitate further optimization to enhance recovery rates.
Case Studies
Chemical Reactions Analysis
Formation of Neodymium Citrate Complexes
Neodymium(III) forms multiple complexes with citric acid depending on pH and solution conditions. Key species identified include:
-
NdCit (mononuclear complex)
-
NdHCit (protonated complex)
-
NdL₂ (dimeric complex)
These complexes form through equilibria involving Nd³⁺, H⁺, and citrate ions (Cit³⁻). For example, the equilibrium for Nd³⁺ + Cit³⁻ ⇌ NdCit³⁻ has been extensively studied using potentiometry and spectrophotometry .
Table 1: Stability Constants for Neodymium-Citrate Complexes
Complex | Log β (Stability Constant) | Method |
---|---|---|
NdCit³⁻ | 12.3 ± 0.2 | Potentiometry |
NdL₂⁶⁻ | 24.1 ± 0.3 | Potentiometry |
Nd(HEDTA)(Hcit)²⁻ | 22.15 ± 0.05 | Spectrophotometry |
Ternary Complexation with HEDTA
This compound can form ternary complexes with ligands like HEDTA (1,2-ethylenediaminetetraacetic acid). The primary species identified is Nd(HEDTA)(Hcit)²⁻ , which forms via:
Nd3++H++HEDTA3−+Cit3−⇌Nd(HEDTA)(Hcit)2−
This complex has a stability constant of log β₁₁₁ = 22.15 ± 0.05 , determined via spectrophotometric titrations .
Thermodynamic Analysis
The formation of this compound complexes is entropy-driven. Microcalorimetry studies reveal that:
-
ΔG (Gibbs free energy) : Dominates over enthalpy (ΔH) in complex formation.
-
ΔH : Typically endothermic for protonation steps.
-
TΔS : Provides the primary driving force for complex stability .
Table 2: Thermodynamic Parameters for Nd³⁺-Citrate Complexation
Parameter | Value (kJ/mol) |
---|---|
ΔG | −57.6 ± 1.2 |
ΔH | 15.2 ± 0.8 |
TΔS | −72.8 ± 1.5 |
Leaching and Dissolution Reactions
Key Leaching Conditions :
Analytical Methods for Characterization
-
Potentiometry : Used to determine stability constants (log β) for binary complexes .
-
Spectrophotometry : Confirmed ternary complex formation via isosbestic points and MCR-ALS analysis .
-
XAFS (X-ray Absorption Fine Structure) : Revealed structural changes in Nd³⁺ coordination during complexation .
Comparison of Stability Constants
The stability of this compound complexes varies significantly depending on ligand type and solution conditions:
Table 3: Stability Constant Trends
Ligand Type | Stability Constant (log β) |
---|---|
Citrate (binary) | 12.3–24.1 |
HEDTA (binary) | ~22.0 |
Ternary (HEDTA + Citrate) | 22.15 |
This compound’s reactivity is primarily governed by its ability to form stable chelate complexes, influenced by pH, ligand concentration, and thermodynamic entropy. Its applications in leaching and separation processes highlight its importance in rare-earth element recovery technologies.
Q & A
Basic Research Questions
Q. What are the standard methods for synthesizing and characterizing neodymium citrate in laboratory settings?
this compound is typically synthesized by reacting neodymium salts (e.g., neodymium chloride) with citric acid in aqueous solutions under controlled pH conditions (e.g., pH 8–10). Characterization involves techniques such as X-ray diffraction (XRD) for crystallinity analysis, Fourier-transform infrared spectroscopy (FTIR) to confirm citrate ligand coordination, and inductively coupled plasma mass spectrometry (ICP-MS) for elemental purity verification . Researchers must ensure stoichiometric ratios are maintained during synthesis to avoid byproducts.
Q. How can this compound be separated from coexisting lanthanides like terbium or dysprosium in mixed solutions?
Separation often employs ionic liquid-based aqueous two-phase systems (IL-ATPS). For example, Aliquat 336 (a quaternary ammonium ionic liquid) selectively extracts this compound by forming complexes such as [NdCl₂Cit²⁻·2Aliquat⁺]. Adjusting pH (optimal range: 10–11) and citrate concentration (42 mmol·L⁻¹) enhances selectivity, while distribution coefficients (Log D) are quantified via UV-Vis spectroscopy or ICP-MS .
Q. What analytical techniques are effective for assessing the purity of this compound samples?
Mass spectrometry (MS) is critical for detecting trace rare-earth impurities (e.g., <1.6 atom% total rare-earth content). Purity evaluation must account for recontamination risks during subsampling. Complementary methods include thermogravimetric analysis (TGA) to verify hydration states and X-ray fluorescence (XRF) for bulk composition .
Advanced Research Questions
Q. How do pH and ligand concentration influence the speciation and stability of this compound complexes in aqueous media?
Speciation is pH-dependent: at pH < 3, Nd³+ remains uncomplexed; at pH 5–7, mononuclear complexes (NdCit⁰) dominate; above pH 9, polynuclear species (e.g., [Nd₂Cit₃]³⁻) form. Citrate concentration modulates stability constants, measured via potentiometric titrations or EXAFS spectroscopy. Researchers should validate speciation models using mass balance equations and activity coefficient assumptions .
Q. What methodologies resolve contradictions in extraction efficiency data for this compound across different ionic liquid systems?
Discrepancies arise from variations in IL hydrophobicity, counterion effects, and citrate coordination modes. Systematic comparison requires normalizing distribution ratios (D) to IL concentration and pH. For instance, Aliquat 336 shows a slope of ~2 in Log D vs. Log [IL] plots, indicating two IL molecules participate in extraction. Cross-validation with spectroscopic techniques (e.g., Raman) clarifies complexation mechanisms .
Q. How can thermodynamic modeling improve the design of this compound recovery processes?
Models integrate extraction equilibria, mass balances, and activity coefficients. For example, the extraction reaction: Nd3++2Cit3−+2Aliquat+↔[NdCit2.2Aliquat]+
is validated by comparing experimental Log D values with predicted outcomes. Software tools (e.g., OLI Analyzer) simulate phase behavior under varying temperatures and ionic strengths .
Q. What strategies mitigate interference from transition metals (e.g., Co²⁺, Ni²⁺) during this compound extraction?
Selective masking agents (e.g., EDTA for Co²⁺) or pH adjustments (e.g., >10 to precipitate transition metals as hydroxides) reduce interference. Competitive extraction studies using radiotracers (e.g., ¹⁴²Nd) quantify selectivity ratios, while X-ray absorption near-edge structure (XANES) analysis identifies competing metal-ligand interactions .
Q. Methodological Considerations
Q. How should researchers validate the reproducibility of this compound extraction protocols?
Reproducibility requires strict control of:
- Ionic strength : Use buffer solutions (e.g., 0.1 M NaCl).
- Phase volume ratios : Maintain 1:1 organic-to-aqueous phase ratios.
- Temperature : Conduct extractions at 25±0.5°C. Triplicate experiments with statistical analysis (e.g., RSD <5%) and interlaboratory comparisons are essential .
Q. What statistical approaches are recommended for analyzing conflicting data on this compound’s coordination geometry?
Apply principal component analysis (PCA) to spectroscopic datasets (e.g., FTIR, XRD) to identify outlier measurements. Pair with density functional theory (DFT) calculations to predict stable geometries. Conflicting results may arise from hydration effects, which are addressed by conducting experiments under inert atmospheres .
Q. How can advanced imaging techniques (e.g., TEM, AFM) elucidate the morphological changes in this compound under varying synthesis conditions?
Transmission electron microscopy (TEM) reveals nanoparticle aggregation at pH > 10, while atomic force microscopy (AFM) quantifies surface roughness changes during dehydration. Coupled with energy-dispersive X-ray spectroscopy (EDS), these techniques map elemental distribution and citrate ligand packing .
Properties
CAS No. |
3002-54-8 |
---|---|
Molecular Formula |
C6H5NdO7 |
Molecular Weight |
333.34 g/mol |
IUPAC Name |
2-hydroxypropane-1,2,3-tricarboxylate;neodymium(3+) |
InChI |
InChI=1S/C6H8O7.Nd/c7-3(8)1-6(13,5(11)12)2-4(9)10;/h13H,1-2H2,(H,7,8)(H,9,10)(H,11,12);/q;+3/p-3 |
InChI Key |
HSULNFGSJFUXON-UHFFFAOYSA-K |
Canonical SMILES |
C(C(=O)[O-])C(CC(=O)[O-])(C(=O)[O-])O.[Nd+3] |
Origin of Product |
United States |
Disclaimer and Information on In-Vitro Research Products
Please be aware that all articles and product information presented on BenchChem are intended solely for informational purposes. The products available for purchase on BenchChem are specifically designed for in-vitro studies, which are conducted outside of living organisms. In-vitro studies, derived from the Latin term "in glass," involve experiments performed in controlled laboratory settings using cells or tissues. It is important to note that these products are not categorized as medicines or drugs, and they have not received approval from the FDA for the prevention, treatment, or cure of any medical condition, ailment, or disease. We must emphasize that any form of bodily introduction of these products into humans or animals is strictly prohibited by law. It is essential to adhere to these guidelines to ensure compliance with legal and ethical standards in research and experimentation.