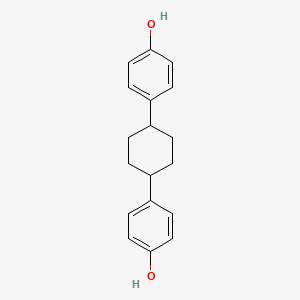
4,4-cyclohexylidene bisphenol
- Click on QUICK INQUIRY to receive a quote from our team of experts.
- With the quality product at a COMPETITIVE price, you can focus more on your research.
Overview
Description
Preparation Methods
Synthetic Routes and Reaction Conditions
4,4-Cyclohexylidene bisphenol is typically synthesized through the condensation reaction of cyclohexanone with phenol. The reaction is catalyzed by an acid, such as sulfuric acid, and involves heating the reactants to facilitate the formation of the bisphenol compound . The general reaction scheme is as follows:
Cyclohexanone+2PhenolH2SO44,4-Cyclohexylidene bisphenol+Water
Industrial Production Methods
In industrial settings, the production of this compound involves similar reaction conditions but on a larger scale. The process is optimized for higher yields and purity, often employing continuous flow reactors and advanced purification techniques such as recrystallization and distillation .
Chemical Reactions Analysis
Types of Reactions
4,4-Cyclohexylidene bisphenol undergoes various chemical reactions, including:
Oxidation: The compound can be oxidized to form quinones.
Reduction: Reduction reactions can convert it into cyclohexylidene derivatives.
Substitution: Electrophilic aromatic substitution reactions can introduce various functional groups onto the aromatic rings.
Common Reagents and Conditions
Oxidation: Common oxidizing agents include potassium permanganate (KMnO4) and chromium trioxide (CrO3).
Reduction: Reducing agents such as sodium borohydride (NaBH4) and lithium aluminum hydride (LiAlH4) are used.
Substitution: Reagents like halogens (Cl2, Br2) and nitrating agents (HNO3) are employed under acidic conditions.
Major Products
Oxidation: Quinones and hydroquinones.
Reduction: Cyclohexylidene derivatives.
Substitution: Halogenated and nitrated bisphenol derivatives.
Scientific Research Applications
4,4-Cyclohexylidene bisphenol has a wide range of applications in scientific research:
Mechanism of Action
The mechanism of action of 4,4-cyclohexylidene bisphenol involves its interaction with molecular targets such as estrogen receptors. It can bind to these receptors and modulate their activity, influencing various biological pathways. The compound’s structural similarity to bisphenol A allows it to mimic estrogenic activity, which is the basis for its use in biological and medical research .
Comparison with Similar Compounds
Similar Compounds
Bisphenol A (BPA): C15H16O2
Bisphenol F (BPF): C13H12O2
Bisphenol S (BPS): C12H10O4S
Bisphenol AF (BPAF): C15H10F6O2
Uniqueness
4,4-Cyclohexylidene bisphenol is unique due to its cyclohexylidene group, which imparts different physical and chemical properties compared to other bisphenols. For instance, it has a higher melting point and different solubility characteristics, making it suitable for specific industrial applications where other bisphenols may not be as effective .
Properties
Molecular Formula |
C18H20O2 |
---|---|
Molecular Weight |
268.3 g/mol |
IUPAC Name |
4-[4-(4-hydroxyphenyl)cyclohexyl]phenol |
InChI |
InChI=1S/C18H20O2/c19-17-9-5-15(6-10-17)13-1-2-14(4-3-13)16-7-11-18(20)12-8-16/h5-14,19-20H,1-4H2 |
InChI Key |
WZCQOMUGRAWORP-UHFFFAOYSA-N |
Canonical SMILES |
C1CC(CCC1C2=CC=C(C=C2)O)C3=CC=C(C=C3)O |
Origin of Product |
United States |
Synthesis routes and methods
Procedure details
Disclaimer and Information on In-Vitro Research Products
Please be aware that all articles and product information presented on BenchChem are intended solely for informational purposes. The products available for purchase on BenchChem are specifically designed for in-vitro studies, which are conducted outside of living organisms. In-vitro studies, derived from the Latin term "in glass," involve experiments performed in controlled laboratory settings using cells or tissues. It is important to note that these products are not categorized as medicines or drugs, and they have not received approval from the FDA for the prevention, treatment, or cure of any medical condition, ailment, or disease. We must emphasize that any form of bodily introduction of these products into humans or animals is strictly prohibited by law. It is essential to adhere to these guidelines to ensure compliance with legal and ethical standards in research and experimentation.