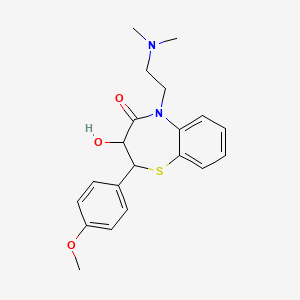
Desacetyl Diltiazem
Overview
Description
Desacetyl Diltiazem is a chemical compound known for its pharmacological properties. It is commonly used as a calcium channel blocker and vasodilator, making it valuable in the treatment of cardiovascular disorders such as angina pectoris and hypertension .
Preparation Methods
Synthetic Routes and Reaction Conditions
The synthesis of Desacetyl Diltiazem typically involves the condensation of β-diethylaminoethyl chloride with 2-(4-methoxyphenyl)-3-hydroxy-2,3-dihydro-1,5-benzothiazepin-4(5H)-one. This is followed by acetylation using acetic anhydride .
Industrial Production Methods
Industrial production of this compound involves similar synthetic routes but on a larger scale, with optimized reaction conditions to ensure high yield and purity. The process includes careful control of temperature, pressure, and the use of catalysts to enhance reaction efficiency .
Chemical Reactions Analysis
Types of Reactions
Desacetyl Diltiazem undergoes various chemical reactions, including:
Oxidation: This reaction can lead to the formation of corresponding oxides.
Reduction: Reduction reactions can convert the compound into its reduced forms.
Substitution: Common substitution reactions involve the replacement of functional groups with others, such as halogenation or alkylation
Common Reagents and Conditions
Oxidation: Reagents like potassium permanganate or hydrogen peroxide under acidic or basic conditions.
Reduction: Reagents such as lithium aluminum hydride or sodium borohydride.
Substitution: Reagents like halogens (chlorine, bromine) or alkylating agents under appropriate conditions
Major Products
The major products formed from these reactions depend on the specific reagents and conditions used. For example, oxidation may yield oxides, while substitution reactions can produce various derivatives with different functional groups .
Scientific Research Applications
Desacetyl Diltiazem has several scientific research applications:
Chemistry: Used as a model compound in studying reaction mechanisms and synthetic methodologies.
Biology: Investigated for its effects on cellular calcium channels and its role in cellular signaling pathways.
Medicine: Widely used in the development of drugs for treating cardiovascular diseases due to its calcium channel blocking properties.
Industry: Employed in the formulation of pharmaceutical products and as an intermediate in the synthesis of other compounds .
Mechanism of Action
The compound exerts its effects by reducing the transmembrane influx of calcium ions into cells of cardiac muscle and smooth musculature of vessels. This leads to the dilation of coronary and peripheral vessels, increased coronary blood flow, and prevention of coronary artery spasms. The molecular targets include calcium channels, and the pathways involved are related to calcium signaling and vascular smooth muscle relaxation .
Comparison with Similar Compounds
Similar Compounds
Verapamil: Another calcium channel blocker with similar vasodilatory effects.
Nifedipine: A dihydropyridine calcium channel blocker used for similar indications.
Amlodipine: A long-acting calcium channel blocker used in the treatment of hypertension and angina
Uniqueness
Desacetyl Diltiazem is unique due to its specific chemical structure, which provides a distinct pharmacokinetic profile and therapeutic efficacy. Its ability to selectively inhibit calcium influx and its favorable side effect profile make it a valuable compound in clinical practice .
Properties
Molecular Formula |
C20H24N2O3S |
---|---|
Molecular Weight |
372.5 g/mol |
IUPAC Name |
5-[2-(dimethylamino)ethyl]-3-hydroxy-2-(4-methoxyphenyl)-2,3-dihydro-1,5-benzothiazepin-4-one |
InChI |
InChI=1S/C20H24N2O3S/c1-21(2)12-13-22-16-6-4-5-7-17(16)26-19(18(23)20(22)24)14-8-10-15(25-3)11-9-14/h4-11,18-19,23H,12-13H2,1-3H3 |
InChI Key |
NZHUXMZTSSZXSB-UHFFFAOYSA-N |
Canonical SMILES |
CN(C)CCN1C2=CC=CC=C2SC(C(C1=O)O)C3=CC=C(C=C3)OC |
Origin of Product |
United States |
Synthesis routes and methods
Procedure details
Disclaimer and Information on In-Vitro Research Products
Please be aware that all articles and product information presented on BenchChem are intended solely for informational purposes. The products available for purchase on BenchChem are specifically designed for in-vitro studies, which are conducted outside of living organisms. In-vitro studies, derived from the Latin term "in glass," involve experiments performed in controlled laboratory settings using cells or tissues. It is important to note that these products are not categorized as medicines or drugs, and they have not received approval from the FDA for the prevention, treatment, or cure of any medical condition, ailment, or disease. We must emphasize that any form of bodily introduction of these products into humans or animals is strictly prohibited by law. It is essential to adhere to these guidelines to ensure compliance with legal and ethical standards in research and experimentation.