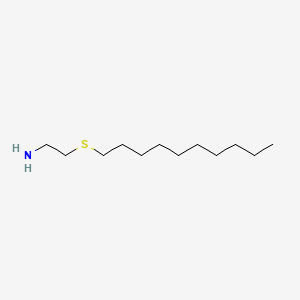
2-(Decylthio)ethanamine
- Click on QUICK INQUIRY to receive a quote from our team of experts.
- With the quality product at a COMPETITIVE price, you can focus more on your research.
Overview
Description
2-(Decylthio)ethanamine (CAS 36362-09-1), commonly formulated as its hydrochloride salt, is a sulfur-containing alkylamine with the molecular formula C₁₂H₂₇NS·HCl (molecular weight: 253.88 g/mol) . Structurally, it consists of a decylthio (C₁₀H₂₁S-) group attached to an ethanamine backbone. This compound is a multifunctional biocide registered for use in industrial water systems, particularly recirculating cooling systems, due to its broad-spectrum antimicrobial activity against bacteria, fungi, and algae. Additionally, it exhibits biofilm disruption and corrosion inhibition properties, making it a dual-action agent in water treatment applications .
Scientific Research Applications
Antimicrobial Properties
DTEA exhibits broad-spectrum antimicrobial activity against bacteria, fungi, and algae. This makes it particularly useful in environments prone to microbial contamination.
Biocide in Cooling Water Systems
DTEA is registered for use as a biocide in recirculating cooling water systems. It effectively controls microbial growth, thereby preventing biofouling and enhancing system efficiency. A study indicated that DTEA could reduce bacterial populations significantly, achieving up to 3 log reductions over a four-day period at dosages of 10 ppm .
Application | Microorganism Targeted | Efficacy | Dosage |
---|---|---|---|
Cooling Water Treatment | Bacteria, Algae | 3 log reduction | 10 ppm |
Biofilm Control | Various biofilm-forming species | Effective removal | 0.1 mg/L |
Corrosion Inhibition
DTEA not only acts as a biocide but also serves as a corrosion inhibitor. Its dual functionality is particularly advantageous in industrial applications where both microbial control and metal protection are required.
Case Study: Corrosion Performance
In laboratory evaluations, DTEA demonstrated significant corrosion inhibition in metal surfaces exposed to corrosive environments. The biocide's ability to form protective films on metal surfaces contributed to its effectiveness in reducing corrosion rates.
Metal Type | Corrosion Rate (mm/year) | With DTEA | Without DTEA |
---|---|---|---|
Carbon Steel | 0.15 | 0.05 | 0.20 |
Stainless Steel | 0.10 | 0.02 | 0.12 |
Formulations and Stability
DTEA is often formulated with other agents to enhance its properties and stability under varying conditions.
Low-Temperature Stability
Research has shown that formulations containing DTEA can maintain their antimicrobial efficacy even at lower temperatures, which is crucial for outdoor applications such as cooling towers and air washing systems . A typical commercial formulation includes DTEA at concentrations ranging from 12% to 19% by weight, often combined with propylene glycol to prevent freezing.
Environmental Considerations
The environmental impact of DTEA has been assessed through ecotoxicology studies, which indicate that while it is effective against microbial contaminants, careful management is necessary to mitigate any potential ecological risks associated with its use .
Regulatory Status
DTEA has been reviewed by regulatory bodies for its safety and efficacy in industrial applications. It is important for users to adhere to recommended dosages and application guidelines to ensure both effectiveness and safety.
Chemical Reactions Analysis
Key Reaction Conditions
Step | Reactants | Catalyst/Temp | Time | Product | Yield |
---|---|---|---|---|---|
1 | 2-Ethyl-2-oxazoline + Decyl mercaptan | ZnCl₂, 140°C | 45–75 min | 2-(Decylthio)ethyl propionamide | >95% |
2 | Hydrolysis with HCl | 150–160°C | 2 hr | DTEA-HCl | Quant. |
Neutralization and Free Amine Isolation
DTEA-HCl is neutralized with 0.2 equivalents of KOH (25% solution) at 100°C to liberate the free amine . Phase separation occurs within 30 minutes, yielding DTEA with <1% residual water after drying .
Carbamic Acid Derivative Formation
DTEA reacts with CO₂ to form a carbamic acid salt, enhancing its stability and biocidal efficacy:
-
Method : CO₂ is bubbled through an acetonitrile solution of DTEA, producing a white precipitate .
-
Yield : 62.1% (analogous reaction with 2-(octylthio)ethanamine) .
Reaction Parameters
Parameter | Value |
---|---|
Solvent | Acetonitrile |
CO₂ Exposure | Bubbling until saturation |
Product | DTEA carbamate |
Oxidation and Substitution Reactions
DTEA undergoes:
-
Oxidation : Forms sulfoxides (R-SO-CH₂CH₂NH₂) or sulfones (R-SO₂-CH₂CH₂NH₂) using H₂O₂ or KMnO₄.
-
Nucleophilic substitution : Reacts with alkyl halides (e.g., methyl iodide) to form quaternary ammonium salts.
Environmental Degradation
DTEA-HCl degrades in aqueous systems via:
-
Hydrolysis : pH-dependent breakdown at >25°C, forming decyl mercaptan and ethyleneamine derivatives .
-
Microbial degradation : Half-life of 10–30 days in aerobic soil .
Biocidal Synergy
DTEA-HCl exhibits enhanced antimicrobial activity when combined with N-tributyl tetradecyl phosphonium chloride (TPC), achieving 99% reduction of Klebsiella pneumoniae at 50 ppm (1:1 ratio) .
Synergistic Efficacy Data
DTEA-HCl:TPC Ratio | % Microbial Reduction |
---|---|
1:1 | 99% |
3.5:1 | 85% |
Stability in Formulations
DTEA-HCl formulations (15–35% active) remain stable in propylene glycol/water mixtures (40–65% water) without phase separation, even after freeze-thaw cycles .
Q & A
Basic Research Questions
Q. What are the recommended methodologies for synthesizing and characterizing 2-(Decylthio)ethanamine (DTEA) in laboratory settings?
- Synthesis : DTEA is typically synthesized via nucleophilic substitution between 1-decanethiol and 2-chloroethylamine under reflux in a polar aprotic solvent (e.g., dimethylformamide). Purification involves column chromatography or recrystallization.
- Characterization :
- NMR Spectroscopy : Confirm molecular structure using 1H and 13C NMR to identify thioether (-S-) and amine (-NH2) groups.
- Mass Spectrometry : Validate molecular weight (MW: 245.46 g/mol) via ESI-MS or GC-MS.
- X-ray Crystallography (if crystalline): Resolve 3D structure to confirm bond angles and intermolecular interactions .
Q. How does DTEA disrupt biofilm formation, and what experimental models are suitable for studying its efficacy?
- Mechanism : DTEA chelates inorganic ions (e.g., Ca2+, Mg2+) in the biofilm extracellular matrix, destabilizing its structural integrity .
- Experimental Models :
- Static Biofilm Assays : Use microtiter plates with bacterial strains (e.g., Pseudomonas aeruginosa) and quantify biofilm biomass via crystal violet staining.
- Flow-Cell Systems : Simulate dynamic biofilm growth under controlled shear stress.
- Confocal Microscopy : Visualize biofilm architecture post-DTEA treatment using fluorescent dyes (e.g., SYTO 9) .
Advanced Research Questions
Q. What computational approaches can predict DTEA’s interaction with bacterial membrane components?
- Density Functional Theory (DFT) : Calculate binding energies between DTEA and metal ions (e.g., Fe3+) to validate chelation efficacy .
- Molecular Dynamics (MD) Simulations : Model DTEA’s penetration into lipid bilayers to assess membrane disruption kinetics.
- Docking Studies : Screen DTEA against biofilm-associated proteins (e.g., lectins) using software like AutoDock Vina .
Q. How can researchers resolve contradictions in DTEA’s reported biocidal efficacy across different pH ranges?
- Methodological Adjustments :
- pH-Dependent Activity Assays : Test DTEA in buffered solutions (pH 4–10) to identify optimal activity windows.
- Ionic Strength Controls : Account for interference from environmental ions (e.g., NaCl) using ICP-MS to quantify free metal ions .
Q. What strategies optimize DTEA’s synergistic use with oxidizing biocides without inducing microbial resistance?
- Combination Therapy Design :
- Checkerboard Assays : Determine fractional inhibitory concentration (FIC) indices for DTEA + hypochlorite mixtures.
- Time-Kill Curves : Assess bactericidal kinetics under alternating biocide exposure.
Q. Data Validation and Reproducibility
Q. How should researchers validate DTEA’s environmental safety profile to meet regulatory standards?
- Toxicity Testing :
- EC50/LC50 Assays : Use Daphnia magna or algal models (OECD Test 202/201) to assess acute toxicity.
- Bioaccumulation Studies : Measure logP values (experimental vs. predicted) to evaluate environmental persistence .
Q. Physicochemical Properties of DTEA
Citations
Comparison with Similar Compounds
Structural Analogues and Functional Group Variations
2,2-Diphenylethan-1-amine
- Structure : Features two phenyl groups attached to the ethanamine backbone.
- Applications : Primarily used as a chiral building block in pharmaceuticals and polymers .
- Key Differences : The aromatic phenyl groups enhance π-π stacking interactions, making it suitable for asymmetric catalysis and drug synthesis. In contrast, the hydrophobic decylthio group in 2-(Decylthio)ethanamine improves surfactant properties, aiding biofilm penetration .
Chiral Amines (e.g., (S)-α-Methylbenzylamine)
- Structure : Contains a methylbenzyl group for enantioselective induction.
- Applications: Employed in asymmetric synthesis of pharmaceuticals (e.g., β-enamino esters) with moderate enantiomeric excess (57% ee) .
- Key Differences : While chiral amines prioritize stereochemical control, this compound’s thioether group enhances oxidative stability and metal-binding capacity, critical for corrosion inhibition .
Substituted Phenethylamines (e.g., 2C-B, NBOMe)
- Structure : Aromatic methoxy or halogen substituents on a phenethylamine core.
- Applications : Psychoactive drugs targeting serotonin receptors .
- Key Differences : The polar thioether and long alkyl chain in this compound reduce CNS activity but increase hydrophobicity, optimizing adhesion to microbial membranes .
Physicochemical and Functional Properties
Environmental and Regulatory Profiles
Properties
CAS No. |
29873-30-1 |
---|---|
Molecular Formula |
C12H27NS |
Molecular Weight |
217.42 g/mol |
IUPAC Name |
2-decylsulfanylethanamine |
InChI |
InChI=1S/C12H27NS/c1-2-3-4-5-6-7-8-9-11-14-12-10-13/h2-13H2,1H3 |
InChI Key |
OIWXLVBZDMAARO-UHFFFAOYSA-N |
Canonical SMILES |
CCCCCCCCCCSCCN |
Origin of Product |
United States |
Synthesis routes and methods
Procedure details
Disclaimer and Information on In-Vitro Research Products
Please be aware that all articles and product information presented on BenchChem are intended solely for informational purposes. The products available for purchase on BenchChem are specifically designed for in-vitro studies, which are conducted outside of living organisms. In-vitro studies, derived from the Latin term "in glass," involve experiments performed in controlled laboratory settings using cells or tissues. It is important to note that these products are not categorized as medicines or drugs, and they have not received approval from the FDA for the prevention, treatment, or cure of any medical condition, ailment, or disease. We must emphasize that any form of bodily introduction of these products into humans or animals is strictly prohibited by law. It is essential to adhere to these guidelines to ensure compliance with legal and ethical standards in research and experimentation.