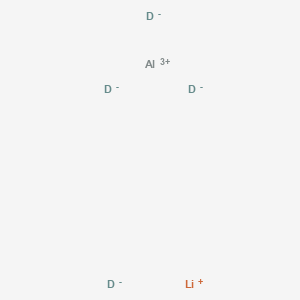
Lithium aluminium deuteride
- Click on QUICK INQUIRY to receive a quote from our team of experts.
- With the quality product at a COMPETITIVE price, you can focus more on your research.
Overview
Description
Aluminum lithium deuteride is a chemical compound that consists of aluminum, lithium, and deuterium atoms. It is a derivative of lithium aluminum hydride, where hydrogen atoms are replaced by deuterium. This compound is known for its use as a reducing agent in organic synthesis and as a source of deuterium atoms in various applications.
Preparation Methods
Synthetic Routes and Reaction Conditions: The synthesis of aluminum lithium deuteride typically involves the reaction of lithium aluminum hydride with lithium deuteride. One common method is ball milling lithium aluminum hydride with lithium deuteride to achieve high purity . Another method involves the reaction of lithium deuteride with aluminum trichloride in an ether solution under reflux conditions . The reaction is carried out at temperatures between 25 to 35 degrees Celsius for several hours to obtain the desired product .
Industrial Production Methods: Industrial production of aluminum lithium deuteride often employs high-temperature deuteration methods. This involves the direct chemical reaction between metals and deuterium gas at elevated temperatures and pressures . The process is advantageous due to its simplicity and the high purity of the resulting product.
Chemical Reactions Analysis
Types of Reactions: Aluminum lithium deuteride undergoes various types of chemical reactions, including reduction, substitution, and decomposition. It is a powerful reducing agent and is commonly used to reduce carbonyl compounds to alcohols .
Common Reagents and Conditions: Common reagents used in reactions with aluminum lithium deuteride include carbonyl compounds, esters, and amides. The reactions are typically carried out in anhydrous conditions to prevent the compound from reacting with water, which can lead to the release of flammable gases .
Major Products Formed: The major products formed from reactions involving aluminum lithium deuteride include alcohols, amines, and hydrocarbons. For example, the reduction of esters with aluminum lithium deuteride yields primary alcohols .
Scientific Research Applications
Aluminum lithium deuteride has a wide range of scientific research applications. It is used as a source of deuterium atoms in the production of tritium for nuclear reactors . In the field of organic synthesis, it is employed as a reducing agent to facilitate the conversion of carbonyl compounds into alcohols .
Mechanism of Action
The mechanism of action of aluminum lithium deuteride involves a series of phase transitions and reactions with electrolytes during discharge and recharge processes . During these processes, lithium aluminum deuteride undergoes structural changes, forming lithium hydride and aluminum. The compound’s high initial capacity and low coulombic efficiency are attributed to irreversible processes during cycling .
Comparison with Similar Compounds
Similar Compounds: Similar compounds to aluminum lithium deuteride include lithium aluminum hydride, sodium aluminum deuteride, and potassium aluminum deuteride . These compounds share similar chemical properties and applications but differ in their specific reactivity and stability.
Uniqueness: Aluminum lithium deuteride is unique due to its high deuterium content, making it particularly valuable as a source of deuterium atoms in various applications. Its ability to act as a powerful reducing agent and its potential use in hydrogen storage further distinguish it from other similar compounds .
Properties
Molecular Formula |
AlH4Li |
---|---|
Molecular Weight |
42.0 g/mol |
IUPAC Name |
aluminum;lithium;deuteride |
InChI |
InChI=1S/Al.Li.4H/q+3;+1;4*-1/i;;4*1+1 |
InChI Key |
BJKLPLABXHXMIM-HGZFDWPVSA-N |
Isomeric SMILES |
[2H-].[2H-].[2H-].[2H-].[Li+].[Al+3] |
Canonical SMILES |
[H-].[H-].[H-].[H-].[Li+].[Al+3] |
Origin of Product |
United States |
Disclaimer and Information on In-Vitro Research Products
Please be aware that all articles and product information presented on BenchChem are intended solely for informational purposes. The products available for purchase on BenchChem are specifically designed for in-vitro studies, which are conducted outside of living organisms. In-vitro studies, derived from the Latin term "in glass," involve experiments performed in controlled laboratory settings using cells or tissues. It is important to note that these products are not categorized as medicines or drugs, and they have not received approval from the FDA for the prevention, treatment, or cure of any medical condition, ailment, or disease. We must emphasize that any form of bodily introduction of these products into humans or animals is strictly prohibited by law. It is essential to adhere to these guidelines to ensure compliance with legal and ethical standards in research and experimentation.