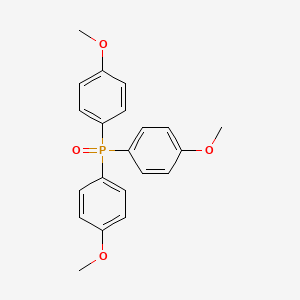
Tris(4-methoxyphenyl)phosphine oxide
- Click on QUICK INQUIRY to receive a quote from our team of experts.
- With the quality product at a COMPETITIVE price, you can focus more on your research.
Overview
Description
Tris(4-methoxyphenyl)phosphine oxide is a useful research compound. Its molecular formula is C21H21O4P and its molecular weight is 368.4 g/mol. The purity is usually 95%.
BenchChem offers high-quality this compound suitable for many research applications. Different packaging options are available to accommodate customers' requirements. Please inquire for more information about this compound including the price, delivery time, and more detailed information at info@benchchem.com.
Scientific Research Applications
Applications in Chemistry
Tris(4-methoxyphenyl)phosphine oxide is predominantly used as a ligand in various catalytic processes:
- Catalytic Reactions : It plays a crucial role in facilitating reactions such as:
- Suzuki-Miyaura Coupling : This reaction is vital for forming carbon-carbon bonds, particularly in the synthesis of biaryl compounds.
- Heck Reaction : Employed in the synthesis of alkenes from aryl halides and alkenes.
- Stille Coupling : Utilized for constructing complex organic molecules through the coupling of organotin compounds with halides.
Table 1: Summary of Catalytic Reactions Involving this compound
Reaction Type | Application | Reference |
---|---|---|
Suzuki-Miyaura | Formation of biaryl compounds | |
Heck | Synthesis of alkenes | |
Stille | Coupling of organotin compounds |
Applications in Biology and Medicine
In biological research, this compound has been investigated for its potential therapeutic applications:
- Enzyme Mechanisms : It serves as a probe for studying enzyme mechanisms and protein-ligand interactions, enhancing the understanding of biochemical pathways.
- Drug Delivery Systems : Research indicates its utility in drug delivery systems due to its ability to form stable complexes with metal ions, which can be utilized to improve the bioavailability of pharmaceuticals.
Case Study: Anticancer Activity
Recent studies have explored the anticancer properties of derivatives related to this compound. For instance, a compound exhibiting potent anti-cancer activity was found to inhibit cell cycle progression effectively against various cancer cell lines, including glioblastoma .
Industrial Applications
This compound is also employed in various industrial processes:
- Production of Fine Chemicals : Its role as a ligand facilitates the synthesis of high-value chemicals and active pharmaceutical ingredients (APIs).
- Stabilizer for Peroxides : The compound is used as a stabilizer in the production processes involving peroxides, enhancing safety and efficiency.
Table 2: Industrial Applications of this compound
Application | Description | Reference |
---|---|---|
Fine Chemical Production | Used as a ligand in synthesizing APIs | |
Stabilizer for Peroxides | Enhances safety during peroxide production |
Chemical Reactions Analysis
Electrochemical Reduction to Triarylphosphine
The P(V) center in triarylphosphine oxides can be electrochemically reduced to P(III) phosphines using triaryl borate Lewis acids. TMTPP reduction with tris(4-methoxyphenyl)borate achieves 37% faradaic efficiency , the highest among tested borates .
Reaction Mechanism :
-
Formation of a two-electron-reduced association complex.
-
Rate-determining phosphoryl bond cleavage.
Triaryl Borate | Faradaic Efficiency |
---|---|
Tris(4-methoxyphenyl)borate | 37% |
Triphenylborate | Lower |
Oxidation of Tris(4-methoxyphenyl)phosphine
Tris(4-methoxyphenyl)phosphine oxide is prepared via oxidation of the parent phosphine using oxalyl chloride and HEH (hydroxylamine hydrochloride) .
Procedure :
-
Oxalyl chloride (0.4 mmol) is added to a solution of the phosphine oxide (0.4 mmol) in CH₂Cl₂.
-
HEH (1 mmol) and TEA (3 mmol) are introduced, followed by stirring at 40°C.
-
Workup involves aqueous extraction and column chromatography .
Hydrodearylation of Triarylphosphine Oxides
Triarylphosphine oxides undergo hydrodearylation using NaH and LiI in THF to form secondary phosphines .
Example Reaction :
-
Reagents : NaH (2–3 equiv), LiI (1–2 equiv), THF, 60°C.
Reduction of Oxide Impurities in Organic Reactions
In processes like Mitsunobu or Wittig reactions, residual triarylphosphine oxides can be reduced using:
Example Protocol :
Functionalization via Electrophilic Substitution
Triarylphosphine oxides undergo dearylation and electrophilic substitution to form functionalized derivatives .
Procedure :
-
Mix phosphine oxide (0.5 mmol), NaH (2–3 equiv), and LiI (1–2 equiv) in THF.
-
Stir at 60°C, then add an electrophile (e.g., benzyl bromide).
Product Example : Benzyldiphenylphosphine oxide (4af) with 79% yield .
Properties
CAS No. |
803-17-8 |
---|---|
Molecular Formula |
C21H21O4P |
Molecular Weight |
368.4 g/mol |
IUPAC Name |
1-bis(4-methoxyphenyl)phosphoryl-4-methoxybenzene |
InChI |
InChI=1S/C21H21O4P/c1-23-16-4-10-19(11-5-16)26(22,20-12-6-17(24-2)7-13-20)21-14-8-18(25-3)9-15-21/h4-15H,1-3H3 |
InChI Key |
KSTMQPBYFFFVFI-UHFFFAOYSA-N |
Canonical SMILES |
COC1=CC=C(C=C1)P(=O)(C2=CC=C(C=C2)OC)C3=CC=C(C=C3)OC |
Origin of Product |
United States |
Synthesis routes and methods
Procedure details
Disclaimer and Information on In-Vitro Research Products
Please be aware that all articles and product information presented on BenchChem are intended solely for informational purposes. The products available for purchase on BenchChem are specifically designed for in-vitro studies, which are conducted outside of living organisms. In-vitro studies, derived from the Latin term "in glass," involve experiments performed in controlled laboratory settings using cells or tissues. It is important to note that these products are not categorized as medicines or drugs, and they have not received approval from the FDA for the prevention, treatment, or cure of any medical condition, ailment, or disease. We must emphasize that any form of bodily introduction of these products into humans or animals is strictly prohibited by law. It is essential to adhere to these guidelines to ensure compliance with legal and ethical standards in research and experimentation.