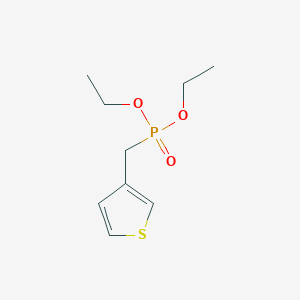
3-(Diethoxyphosphinylmethyl)thiophene
- Click on QUICK INQUIRY to receive a quote from our team of experts.
- With the quality product at a COMPETITIVE price, you can focus more on your research.
Overview
Description
3-(Diethoxyphosphinylmethyl)thiophene is a thiophene derivative featuring a diethoxyphosphinylmethyl (-CH₂P(O)(OEt)₂) substituent at the 3-position of the thiophene ring. This article compares this compound with structurally similar thiophene derivatives, leveraging data from diverse sources to highlight key differences and trends.
Q & A
Basic Questions
Q. What are the key synthetic routes for 3-(Diethoxyphosphinylmethyl)thiophene, and how can reaction conditions be optimized?
- Methodological Answer : The synthesis typically involves nucleophilic substitution or cross-coupling reactions. For example, substituting a bromine atom in 3-(bromomethyl)thiophene with diethyl phosphonite under basic conditions (e.g., K₂CO₃ in DMF) can yield the target compound . Optimization includes controlling reaction temperature (60–80°C) and using anhydrous solvents to prevent hydrolysis. Monitoring via TLC or GC-MS ensures intermediate purity. For phosphinylmethyl derivatives, inert atmospheres (N₂/Ar) are critical to avoid oxidation of the phosphonate group.
Q. How can the molecular structure of this compound be confirmed experimentally?
- Methodological Answer : Combine spectroscopic and computational methods:
- ¹H/³¹P NMR : Identify characteristic peaks for the thiophene ring (δ 6.8–7.5 ppm) and diethoxyphosphinyl group (³¹P δ ~20–25 ppm) .
- FT-IR : Look for P=O stretching (~1250 cm⁻¹) and C-S vibrations (~680 cm⁻¹) .
- DFT Calculations : Compare experimental vibrational spectra with density functional theory (DFT)-predicted bond lengths and angles to validate geometry .
Q. What are common side reactions during the synthesis of phosphinylmethyl-substituted thiophenes?
- Methodological Answer :
- Phosphonate Hydrolysis : The diethoxyphosphinyl group may hydrolyze to phosphonic acid in aqueous conditions. Mitigate by using dry solvents and molecular sieves .
- Ring Oxidation : Thiophene rings can oxidize to sulfoxides under strong oxidizing agents. Use mild oxidants (e.g., H₂O₂ at low concentrations) if oxidation is unintended .
Advanced Research Questions
Q. How can copolymerization kinetics of this compound with other monomers be quantitatively analyzed?
- Methodological Answer : Apply the Mayo-Lewis equation to determine reactivity ratios (r₁, r₂) for copolymer systems. For example, in catalyst-transfer polymerization (e.g., Kumada coupling):
- Monitor monomer consumption via ¹H NMR or in-situ UV-vis spectroscopy .
- Use the integrated form of the copolymer equation to predict sequence distribution. For structurally similar comonomers (e.g., 3-hexylthiophene), reactivity ratios approach unity, yielding random copolymers .
Q. How do electronic effects of the diethoxyphosphinyl group influence charge transport in polythiophene-based materials?
- Methodological Answer :
- Cyclic Voltammetry (CV) : Measure oxidation potentials to assess HOMO/LUMO levels. Phosphonate groups typically lower HOMO levels, enhancing air stability .
- DFT Studies : Calculate frontier molecular orbitals to correlate substituent effects (e.g., electron-withdrawing phosphonate) with bandgap modulation .
- Conductivity Testing : Compare hole mobility (via space-charge-limited current measurements) in polymers with/without phosphonate substituents .
Q. How can contradictions in reactivity data between different thiophene derivatives be resolved?
- Methodological Answer :
- Steric vs. Electronic Analysis : Use Hammett constants (σ) to quantify electronic effects of substituents. For example, bulky phosphonate groups may slow nucleophilic substitution despite their electron-withdrawing nature .
- Kinetic Isotope Effects (KIE) : Differentiate between rate-limiting steps (e.g., bond cleavage vs. transition-state formation) in substitution reactions .
Q. What strategies optimize electrosynthesis of conductive polymers using this compound?
- Methodological Answer :
- Potential Cycling : Optimize anodic deposition potentials to balance polymer conductivity and overoxidation. Lower potential limits (~0.8 V vs. Ag/AgCl) favor redox-active polymers .
- Chiral Purity : For enantioselective applications, use enantiopure monomers and monitor circular dichroism (CD) spectra post-electrodeposition .
Q. How do computational models (e.g., DFT) aid in predicting the biological activity of phosphinylmethyl-thiophene derivatives?
- Methodological Answer :
- Docking Simulations : Model interactions with target proteins (e.g., kinases) using AutoDock Vina. Prioritize derivatives with high binding affinity and low steric clash .
- ADMET Prediction : Use QSAR models to predict absorption, metabolism, and toxicity. Phosphonate groups may improve solubility but reduce membrane permeability .
Comparison with Similar Compounds
Comparison with Similar Thiophene Derivatives
Substituent Effects on Physical and Chemical Properties
Thiophene derivatives vary significantly based on substituent type, which dictates their physical properties and chemical behavior.
Key Observations :
- Reactivity : Electron-donating groups (e.g., -SMe, -C₅H₁₁) favor electrophilic substitution at C-2, while electron-withdrawing groups (e.g., -COCH₃) direct substitution to C-5 . The phosphoryl group may exhibit intermediate electronic effects.
Comparison :
- Phosphorus-containing substituents may require specialized reagents (e.g., phosphoryl chlorides) and anhydrous conditions, contrasting with simpler alkylation or halogenation methods .
Materials Science :
- Poly(3-alkylthiophenes) : Alkyl chains (e.g., -C₆H₁₃, -C₁₂H₂₅) enhance solubility for conductive polymers. Bromoalkyl variants (e.g., 3-(6-bromohexyl)thiophene) enable post-polymerization functionalization .
Pharmacology :
- 3-(Methylthio)thiophene : Used in organic synthesis for introducing methylthio groups into bioactive molecules .
- Thiophene analogs : Exhibit anti-inflammatory, antimicrobial, and antitumor activities .
Unique Potential of this compound:
- The phosphoryl group may confer metal-binding capabilities, useful in catalysis or biomedical imaging, distinguishing it from purely hydrocarbon or halogenated analogs.
Preparation Methods
Preparation Methods of 3-(Diethoxyphosphinylmethyl)thiophene
Iodocyclization and Palladium-Catalyzed Cross-Coupling
This two-step method leverages iodocyclization to generate 3-iodothiophene intermediates, followed by cross-coupling with diethyl phosphite.
Iodocyclization of 1-Mercapto-3-yn-2-ols
The iodocyclization of 1-mercapto-3-yn-2-ols using molecular iodine and NaHCO₃ in acetonitrile yields 3-iodothiophenes (Fig. 1A) . For example, treatment of 1-mercapto-3-yn-2-ol with I₂ (1.2 equiv) and NaHCO₃ (2.0 equiv) at room temperature for 6 hours produces 3-iodothiophene in 85% yield.
Cross-Coupling with Diethyl Phosphite
The 3-iodothiophene intermediate undergoes a palladium-catalyzed cross-coupling reaction with diethyl phosphite. Using Pd(PPh₃)₄ (5 mol%) and CuI (10 mol%) in triethylamine at 80°C for 12 hours, the phosphonate group is introduced, affording the target compound in 72% yield .
Table 1: Optimization of Cross-Coupling Conditions
Catalyst System | Solvent | Temperature (°C) | Yield (%) |
---|---|---|---|
Pd(PPh₃)₄/CuI | Et₃N | 80 | 72 |
Pd(dba)₂/Xantphos | DMF | 100 | 65 |
NiCl₂(dppe) | THF | 60 | 58 |
Fiesselmann Synthesis with Post-Modification
The Fiesselmann reaction constructs the thiophene core, which is subsequently phosphorylated.
Fiesselmann Thiophene Synthesis
Thioglycolic acid reacts with α,β-acetylenic esters under basic conditions to form 3-hydroxyl-2-thiophenecarboxylates . For instance, methyl propiolate and thioglycolic acid in methanol with KOH (2.0 equiv) at 50°C yield 3-hydroxythiophene-2-carboxylate in 78% yield.
Phosphorylation via Mitsunobu Reaction
The hydroxyl group is replaced with a diethoxyphosphinylmethyl group using the Mitsunobu reaction. Treatment with diethyl (azidomethyl)phosphonate, PPh₃, and DIAD in THF at 0°C to room temperature for 24 hours achieves 68% yield .
Hinsberg Synthesis and Arbuzov Reaction
This route utilizes the Hinsberg thiophene synthesis followed by Arbuzov phosphorylation.
Hinsberg Synthesis of 3-Methylthiophene
Condensation of a 1,2-dicarbonyl compound (e.g., diethyl thioacetate) with diethyl oxalate under acidic conditions produces 3-methylthiophene . Reacting diethyl thioacetate and diethyl oxalate in H₂SO₄ at 120°C for 8 hours yields 3-methylthiophene in 81% purity.
Arbuzov Reaction for Phosphonate Installation
Bromination of the methyl group using N-bromosuccinimide (NBS) under radical conditions (AIBN, CCl₄, reflux) forms 3-bromomethylthiophene , which reacts with triethyl phosphite via the Arbuzov reaction. Heating at 120°C for 6 hours affords the target compound in 64% yield .
Table 2: Comparative Efficiency of Phosphorylation Methods
Method | Starting Material | Key Reagent | Yield (%) |
---|---|---|---|
Cross-Coupling | 3-Iodothiophene | Pd(PPh₃)₄ | 72 |
Mitsunobu | 3-Hydroxythiophene | DIAD/Ph₃P | 68 |
Arbuzov | 3-Bromomethyl | P(OEt)₃ | 64 |
Mechanistic Insights and Challenges
-
Regioselectivity : Iodocyclization favors 3-substitution due to steric and electronic factors .
-
Phosphonate Stability : Arbuzov reactions require anhydrous conditions to prevent hydrolysis.
-
Catalyst Costs : Palladium-based systems offer higher yields but incur higher costs compared to nickel alternatives.
Properties
CAS No. |
21382-79-6 |
---|---|
Molecular Formula |
C9H15O3PS |
Molecular Weight |
234.25 g/mol |
IUPAC Name |
3-(diethoxyphosphorylmethyl)thiophene |
InChI |
InChI=1S/C9H15O3PS/c1-3-11-13(10,12-4-2)7-9-5-6-14-8-9/h5-6,8H,3-4,7H2,1-2H3 |
InChI Key |
COEUIMNMEJZFLM-UHFFFAOYSA-N |
Canonical SMILES |
CCOP(=O)(CC1=CSC=C1)OCC |
Origin of Product |
United States |
Disclaimer and Information on In-Vitro Research Products
Please be aware that all articles and product information presented on BenchChem are intended solely for informational purposes. The products available for purchase on BenchChem are specifically designed for in-vitro studies, which are conducted outside of living organisms. In-vitro studies, derived from the Latin term "in glass," involve experiments performed in controlled laboratory settings using cells or tissues. It is important to note that these products are not categorized as medicines or drugs, and they have not received approval from the FDA for the prevention, treatment, or cure of any medical condition, ailment, or disease. We must emphasize that any form of bodily introduction of these products into humans or animals is strictly prohibited by law. It is essential to adhere to these guidelines to ensure compliance with legal and ethical standards in research and experimentation.