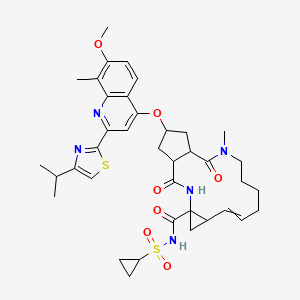
SIMEPREVIR
Overview
Description
N-17-[2-(4-isopropylthiazole-2-yl)-7-methoxy-8-methylquinolin-4-yloxy]-13-methyl-2,14-dioxo-3,13-diazatricyclo[13.3.0.04,6]octadec-7-ene-4-carbonylsulfonamide is a complex organic compound with a unique structure that combines multiple functional groups, including thiazole, quinoline, and sulfonamide moieties
Preparation Methods
Synthetic Routes and Reaction Conditions
The synthesis of N-17-[2-(4-isopropylthiazole-2-yl)-7-methoxy-8-methylquinolin-4-yloxy]-13-methyl-2,14-dioxo-3,13-diazatricyclo[13.3.0.04,6]octadec-7-ene-4-carbonylsulfonamide involves multiple steps, each requiring specific reaction conditions The process typically begins with the preparation of the thiazole and quinoline intermediates, followed by their coupling through a series of condensation and cyclization reactions
Industrial Production Methods
In an industrial setting, the production of this compound would require optimization of the reaction conditions to ensure high yield and purity. This includes controlling the temperature, pressure, and concentration of reactants, as well as employing catalysts to enhance reaction rates. The use of continuous flow reactors and automated synthesis platforms can further improve the efficiency and scalability of the production process.
Chemical Reactions Analysis
Types of Reactions
N-17-[2-(4-isopropylthiazole-2-yl)-7-methoxy-8-methylquinolin-4-yloxy]-13-methyl-2,14-dioxo-3,13-diazatricyclo[13.3.0.04,6]octadec-7-ene-4-carbonylsulfonamide undergoes various chemical reactions, including:
Oxidation: The compound can be oxidized using oxidizing agents such as potassium permanganate or hydrogen peroxide, leading to the formation of sulfoxides or sulfones.
Reduction: Reduction reactions can be carried out using reducing agents like lithium aluminum hydride or sodium borohydride, resulting in the formation of amines or alcohols.
Substitution: The compound can undergo nucleophilic substitution reactions, where nucleophiles such as amines or thiols replace specific functional groups.
Common Reagents and Conditions
Oxidation: Potassium permanganate in acidic or neutral conditions.
Reduction: Lithium aluminum hydride in anhydrous ether.
Substitution: Nucleophiles like amines in the presence of a base such as sodium hydroxide.
Major Products Formed
Oxidation: Sulfoxides, sulfones.
Reduction: Amines, alcohols.
Substitution: Various substituted derivatives depending on the nucleophile used.
Scientific Research Applications
N-17-[2-(4-isopropylthiazole-2-yl)-7-methoxy-8-methylquinolin-4-yloxy]-13-methyl-2,14-dioxo-3,13-diazatricyclo[13.3.0.04,6]octadec-7-ene-4-carbonylsulfonamide has a wide range of applications in scientific research:
Chemistry: Used as a building block for the synthesis of more complex molecules and as a reagent in various organic reactions.
Biology: Investigated for its potential as a bioactive compound with antimicrobial, antiviral, and anticancer properties.
Medicine: Explored for its potential therapeutic applications, including as a drug candidate for treating various diseases.
Industry: Utilized in the development of advanced materials with specific properties, such as conductivity or fluorescence.
Mechanism of Action
The mechanism of action of N-17-[2-(4-isopropylthiazole-2-yl)-7-methoxy-8-methylquinolin-4-yloxy]-13-methyl-2,14-dioxo-3,13-diazatricyclo[13.3.0.04,6]octadec-7-ene-4-carbonylsulfonamide involves its interaction with specific molecular targets, such as enzymes or receptors. The compound’s unique structure allows it to bind to these targets with high affinity, modulating their activity and leading to various biological effects. The pathways involved may include inhibition of enzyme activity, disruption of cellular processes, or modulation of signaling pathways.
Comparison with Similar Compounds
Similar Compounds
- **N-17-[2-(4-isopropylthiazole-2-yl)-7-methoxy-8-methylquinolin-4-yloxy]-13-methyl-2,14-dioxo-3,13-diazatricyclo[13.3.0.04,6]octadec-7-ene-4-carbonylamide
- **N-17-[2-(4-isopropylthiazole-2-yl)-7-methoxy-8-methylquinolin-4-yloxy]-13-methyl-2,14-dioxo-3,13-diazatricyclo[13.3.0.04,6]octadec-7-ene-4-carbonylcarbamate
Uniqueness
The uniqueness of N-17-[2-(4-isopropylthiazole-2-yl)-7-methoxy-8-methylquinolin-4-yloxy]-13-methyl-2,14-dioxo-3,13-diazatricyclo[13.3.0.04,6]octadec-7-ene-4-carbonylsulfonamide lies in its specific combination of functional groups, which confer distinct chemical and biological properties
Properties
Key on ui mechanism of action |
Simeprevir sodium is a selective hepatitis C virus (HCV) nonstructural 3/4A (NS3/4A) protease inhibitor (PI). The drug is a direct-acting antiviral (DAA) with activity against HCV. Simeprevir binds noncovalently to the active site of HCV NS3/4A protease, thereby blocking enzyme activity essential for viral replication (i.e., cleavage of the HCV-encoded polyprotein at the NS3/NS4A, NS4A/NS4B, NS4A/NS5A, and NS5A/NS5B junctions). In vitro studies using biochemical and cell-based replicon assays indicate that simeprevir has potent activity against HCV genotypes 1a and 1b1 5 8 9 and has some activity against HCV genotypes 2, 4, 5, and 6 (not genotype 3). |
---|---|
Molecular Formula |
C38H47N5O7S2 |
Molecular Weight |
749.9 g/mol |
IUPAC Name |
N-cyclopropylsulfonyl-17-[7-methoxy-8-methyl-2-(4-propan-2-yl-1,3-thiazol-2-yl)quinolin-4-yl]oxy-13-methyl-2,14-dioxo-3,13-diazatricyclo[13.3.0.04,6]octadec-7-ene-4-carboxamide |
InChI |
InChI=1S/C38H47N5O7S2/c1-21(2)30-20-51-35(40-30)29-18-32(26-13-14-31(49-5)22(3)33(26)39-29)50-24-16-27-28(17-24)36(45)43(4)15-9-7-6-8-10-23-19-38(23,41-34(27)44)37(46)42-52(47,48)25-11-12-25/h8,10,13-14,18,20-21,23-25,27-28H,6-7,9,11-12,15-17,19H2,1-5H3,(H,41,44)(H,42,46) |
InChI Key |
JTZZSQYMACOLNN-UHFFFAOYSA-N |
SMILES |
CC1=C(C=CC2=C1N=C(C=C2OC3CC4C(C3)C(=O)N(CCCCC=CC5CC5(NC4=O)C(=O)NS(=O)(=O)C6CC6)C)C7=NC(=CS7)C(C)C)OC |
Canonical SMILES |
CC1=C(C=CC2=C1N=C(C=C2OC3CC4C(C3)C(=O)N(CCCCC=CC5CC5(NC4=O)C(=O)NS(=O)(=O)C6CC6)C)C7=NC(=CS7)C(C)C)OC |
Color/Form |
White to almost white powder |
solubility |
Practically insoluble in water over a wide pH range Practically insoluble in propylene glycol; very slightly soluble in ethanol; slightly soluble in acetone. Soluble in dichloromethane; freely soluble in some organic solvents |
Origin of Product |
United States |
Synthesis routes and methods I
Procedure details
Synthesis routes and methods II
Procedure details
Retrosynthesis Analysis
AI-Powered Synthesis Planning: Our tool employs the Template_relevance Pistachio, Template_relevance Bkms_metabolic, Template_relevance Pistachio_ringbreaker, Template_relevance Reaxys, Template_relevance Reaxys_biocatalysis model, leveraging a vast database of chemical reactions to predict feasible synthetic routes.
One-Step Synthesis Focus: Specifically designed for one-step synthesis, it provides concise and direct routes for your target compounds, streamlining the synthesis process.
Accurate Predictions: Utilizing the extensive PISTACHIO, BKMS_METABOLIC, PISTACHIO_RINGBREAKER, REAXYS, REAXYS_BIOCATALYSIS database, our tool offers high-accuracy predictions, reflecting the latest in chemical research and data.
Strategy Settings
Precursor scoring | Relevance Heuristic |
---|---|
Min. plausibility | 0.01 |
Model | Template_relevance |
Template Set | Pistachio/Bkms_metabolic/Pistachio_ringbreaker/Reaxys/Reaxys_biocatalysis |
Top-N result to add to graph | 6 |
Feasible Synthetic Routes
Disclaimer and Information on In-Vitro Research Products
Please be aware that all articles and product information presented on BenchChem are intended solely for informational purposes. The products available for purchase on BenchChem are specifically designed for in-vitro studies, which are conducted outside of living organisms. In-vitro studies, derived from the Latin term "in glass," involve experiments performed in controlled laboratory settings using cells or tissues. It is important to note that these products are not categorized as medicines or drugs, and they have not received approval from the FDA for the prevention, treatment, or cure of any medical condition, ailment, or disease. We must emphasize that any form of bodily introduction of these products into humans or animals is strictly prohibited by law. It is essential to adhere to these guidelines to ensure compliance with legal and ethical standards in research and experimentation.