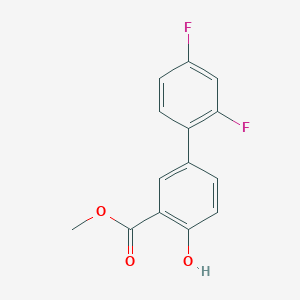
Diflunisal Methyl Ester
Overview
Description
Diflunisal Methyl Ester (methyl 2',4'-difluoro-4-hydroxybiphenyl-3-carboxylate) is a prodrug derivative of the nonsteroidal anti-inflammatory drug (NSAID) diflunisal, designed to improve pharmacokinetic properties while retaining therapeutic efficacy. Synthesized via esterification of the parent compound’s carboxylic acid group (CAS: 55544-00-8) , it exhibits enhanced lipophilicity, which may improve absorption and reduce gastric irritation compared to diflunisal . In vitro studies confirm its stability in acidic conditions (pH 1) but rapid hydrolysis to diflunisal in alkaline environments (pH 10), following pseudo-first-order kinetics . Preliminary in vivo studies in rabbits demonstrate systemic conversion to diflunisal within 3 hours of administration .
Chemical Reactions Analysis
pH-Dependent Stability
The ester bond undergoes hydrolysis in aqueous media, with rates dependent on pH:
Condition | Hydrolysis Rate Constant (k, h⁻¹) | Half-Life (t₁/₂) | Source |
---|---|---|---|
0.1 N HCl (pH 1) | 0.0032 | 216.6 hours | |
pH 7.4 buffer | 0.012 | 57.8 hours | |
pH 10 buffer | 0.056 | 12.4 hours |
Key Observations :
-
Acidic Stability : Minimal hydrolysis at pH 1 due to protonation of the ester carbonyl oxygen, reducing nucleophilic attack .
-
Alkaline Lability : Rapid hydrolysis at pH 10 via base-catalyzed ester cleavage, yielding diflunisal and methanol .
Enzymatic Hydrolysis
In biological matrices (e.g., human serum albumin, HSA):
-
Hydrolysis rates decrease due to protein binding , which sterically shields the ester .
-
Half-life in 2% bovine serum albumin (BSA) at 25°C: 72 hours (vs. 57.8 hours in buffer alone) .
Comparative Hydrolysis Kinetics
Diflunisal methyl ester hydrolyzes faster than its ethyl ester counterpart but slower than aspirin derivatives:
Compound | Relative Hydrolysis Rate (pH 10) | Half-Life (pH 10) |
---|---|---|
This compound | 1.0 (reference) | 12.4 hours |
Diflunisal ethyl ester | 0.67 | 18.5 hours |
Aspirin methyl ester | 3.2 | 3.9 hours |
The slower hydrolysis of diflunisal esters compared to aspirin analogs is attributed to electron-withdrawing fluorine atoms , which reduce electron density at the ester carbonyl, hindering nucleophilic attack .
Photochemical Degradation
Under UV irradiation (254–309 nm), this compound undergoes:
-
Decarboxylation : Loss of CO₂ from the ester group.
-
Defluorination : Cleavage of C-F bonds, generating reactive intermediates .
-
Oxidation : Formation of quinone-like structures via hydroxyl radical scavenging .
Degradation follows first-order kinetics (R² > 0.98), with a half-life of 4.2 hours under simulated sunlight (pH 7.4) .
Biotransformation Pathways
In vivo, this compound is metabolized via:
-
Hepatic Esterases : Rapid conversion to diflunisal, detected in plasma within 3 hours post-administration .
-
CYP450 Oxidation : Minor pathway producing hydroxylated metabolites .
-
Glucuronidation : Formation of acyl glucuronides, which undergo isomerization and covalent adduct formation with proteins .
Nucleophilic Substitution
The methyl ester participates in:
-
Aminolysis : Reaction with amines to form amides (e.g., hydrazides) .
-
Transesterification : Methanol exchange with higher alcohols under acidic conditions .
Cyclization Reactions
Under dehydrating agents (e.g., P₂O₅), the ester facilitates oxazole ring formation via intramolecular cyclization .
Stability in Formulation
-
Solid-State Stability : >24 months at 25°C (no detectable degradation) .
-
Solution Stability : Limited to pH 3–5; precipitates at pH <2 due to poor solubility (0.12 mg/mL) .
Key Research Findings
-
This compound’s hydrolysis half-life in blood (72 hours) supports its use as a prodrug for sustained diflunisal release .
-
Fluorine atoms confer resistance to enzymatic hydrolysis, enhancing metabolic stability compared to non-fluorinated analogs .
-
Photodegradation products exhibit higher toxicity (Toxtree class III) than the parent ester, necessitating UV-protected packaging .
Q & A
Q. Basic: What synthetic methodologies are commonly employed to prepare Diflunisal Methyl Ester, and how are reaction conditions optimized?
Answer: this compound is synthesized via esterification of Diflunisal (a 2,4-difluorobiphenyl derivative) using methanol in the presence of acid or base catalysts. Key parameters include molar ratios of reactants, catalyst type (e.g., sulfuric acid or potassium hydroxide), and reaction temperature. For optimization, factorial design or Taguchi methods can systematically evaluate interactions between variables (e.g., catalyst concentration, temperature) to maximize yield . Characterization typically involves NMR, FT-IR, and HPLC to confirm ester formation and purity .
Q. Basic: What analytical techniques are critical for characterizing this compound in research settings?
Answer: Essential techniques include:
- NMR spectroscopy (¹H/¹³C) to confirm ester bond formation and fluorine substituent positions.
- FT-IR for identifying functional groups (e.g., ester C=O stretch at ~1740 cm⁻¹).
- HPLC-MS for purity assessment and quantification, using reverse-phase columns (C18) with UV detection at 254 nm.
- X-ray crystallography (if crystalline) to resolve stereochemical details .
Q. Advanced: How does the esterification of Diflunisal alter its pharmacological activity compared to the parent compound?
Answer: Methyl esterification modifies Diflunisal’s pharmacokinetics by increasing lipophilicity, potentially enhancing blood-brain barrier penetration. However, ester derivatives may require enzymatic hydrolysis (e.g., esterases) to release the active form. Comparative studies using surface plasmon resonance (SPR) or isothermal titration calorimetry (ITC) can quantify binding affinities to targets like transthyretin, where ester groups may stabilize hydrophobic interactions with Lys15/Thr106 residues .
Q. Advanced: What experimental design strategies are recommended for optimizing large-scale synthesis of this compound while minimizing byproducts?
Answer: Use Taguchi orthogonal arrays (e.g., L9 or L16 designs) to assess interactions between variables like catalyst loading (0.5–1.5 wt%), methanol-to-acid molar ratios (6:1–12:1), and temperature (40–80°C). Response surface methodology (RSM) further refines optimal conditions. Monitor byproducts (e.g., unreacted Diflunisal) via GC-MS or TLC. Continuous flow reactors can enhance reproducibility and reduce side reactions .
Q. Basic: How do researchers validate the identity and purity of this compound in novel synthetic routes?
Answer: Validation requires:
- Chromatographic purity : HPLC with ≥95% area under the curve.
- Elemental analysis : Matching calculated vs. observed C/H/F/O percentages.
- Melting point consistency : Compare with literature values (±2°C).
- Spectroscopic cross-verification : NMR chemical shifts for methyl ester (~3.7 ppm in ¹H) and fluorine atoms .
Q. Advanced: What computational tools are used to predict the stability and reactivity of this compound in physiological environments?
Answer: Molecular dynamics (MD) simulations with software like GROMACS model ester hydrolysis rates in plasma. Density functional theory (DFT) calculations (e.g., B3LYP/6-311G**) predict electron density distributions at ester bonds, guiding structural modifications for enhanced stability. QSAR models correlate logP values with membrane permeability .
Q. Advanced: How can researchers resolve contradictions in reported bioactivity data for this compound across studies?
Answer: Discrepancies may arise from variations in assay conditions (e.g., pH, serum protein content). Strategies include:
- Standardized protocols : Align with guidelines like OECD 428 for in vitro permeability assays.
- Meta-analysis : Pool data from multiple studies using fixed/random-effects models.
- Orthogonal assays : Confirm activity via SPR (binding affinity) and cell-based models (e.g., HEK293 transfected with transthyretin) .
Q. Basic: What are the key considerations for designing in vitro studies to evaluate this compound’s metabolic stability?
Answer: Use liver microsomes (human/rat) or hepatocytes to assess esterase-mediated hydrolysis. Incubate at 37°C with NADPH regeneration systems. Quantify parent compound and metabolites (e.g., Diflunisal) via LC-MS/MS. Control for nonspecific degradation by including esterase inhibitors (e.g., bis-para-nitrophenyl phosphate) in parallel experiments .
Q. Advanced: What role does fluorine substitution play in the physicochemical properties of this compound?
Answer: The 2,4-difluoro groups enhance metabolic stability by reducing cytochrome P450-mediated oxidation. Fluorine’s electronegativity increases hydrogen-bonding potential with target proteins (e.g., transthyretin’s Lys15), as shown in X-ray co-crystallography studies. LogD calculations (at pH 7.4) predict improved membrane permeability compared to non-fluorinated analogs .
Q. Advanced: How can researchers integrate green chemistry principles into the synthesis of this compound?
Answer: Strategies include:
- Solvent selection : Replace dichloromethane with cyclopentyl methyl ether (CPME), a greener solvent.
- Catalyst recycling : Use immobilized lipases (e.g., Candida antarctica Lipase B) for esterification.
- Waste minimization : Employ continuous flow reactors to reduce solvent volume and energy consumption .
Comparison with Similar Compounds
Structural and Physicochemical Properties
Diflunisal Methyl Ester is part of a homologous series of esters (methyl, ethyl, isopropyl, t-butyl) and amide derivatives. Key differences include:
- Crystallization Behavior : Unlike the methyl, ethyl, and isopropyl esters—which form needle crystals due to 1D molecular stacking—the t-butyl ester crystallizes as blocks. This is attributed to steric hindrance from the bulky t-butyl group, preventing efficient stacking .
- Hydrolysis Kinetics : The methyl ester hydrolyzes 2.25× faster than the ethyl ester at pH 10, critical for prodrug activation .
Pharmacological and Metabolic Profiles
- Prodrug Efficiency : Methyl ester’s rapid hydrolysis in alkaline conditions ensures timely release of diflunisal in systemic circulation, whereas the ethyl ester’s slower hydrolysis may delay therapeutic onset .
- Enzyme Interactions : this compound shows reduced allosteric modulation of GABAA receptors compared to diflunisal, suggesting esterification attenuates receptor binding . In contrast, the amide derivative retains partial activity .
- Hepatic Disposition: Hepatic clearance of O-acyl esters correlates with lipophilicity. For example, hexanoyl ester (C6D) exhibits higher hepatic uptake (RN/fu = 16.50) compared to methyl ester (C2D, RN/fu = 9.57) due to enhanced membrane permeability .
Pharmacokinetic Considerations
- Protein Binding : this compound’s unbound fraction in plasma increases in renal failure, altering intrinsic clearance and volume of distribution .
Research Findings and Implications
- Prodrug Optimization : Methyl ester’s balance of stability and hydrolysis kinetics makes it superior to ethyl ester for rapid systemic delivery, while t-butyl derivatives may suit sustained-release applications .
- Clinical Trade-offs : While ester derivatives mitigate diflunisal’s gastric toxicity, their reduced receptor binding (e.g., GABAA) necessitates dose adjustments .
Data Tables
Table 1. Hydrolysis Kinetics of Diflunisal Derivatives (pH 10)
Compound | Rate Constant (k, min⁻¹) | Half-Life (t1/2, min) |
---|---|---|
Methyl Ester | 0.045 | 15.4 |
Amide | 0.030 | 23.1 |
Ethyl Ester | 0.020 | 34.7 |
Table 2. Hepatic Disposition of O-Acyl Esters
Ester | Hepatic Availability (AUC') | RN/fu (Removal Efficiency) |
---|---|---|
Acetyl (C2D) | 0.21 ± 0.03 | 9.57 |
Hexanoyl (C6D) | 0.34 ± 0.05 | 16.50 |
Properties
Molecular Formula |
C14H10F2O3 |
---|---|
Molecular Weight |
264.22 g/mol |
IUPAC Name |
methyl 5-(2,4-difluorophenyl)-2-hydroxybenzoate |
InChI |
InChI=1S/C14H10F2O3/c1-19-14(18)11-6-8(2-5-13(11)17)10-4-3-9(15)7-12(10)16/h2-7,17H,1H3 |
InChI Key |
QMPIMDAYFIIMIH-UHFFFAOYSA-N |
Canonical SMILES |
COC(=O)C1=C(C=CC(=C1)C2=C(C=C(C=C2)F)F)O |
Origin of Product |
United States |
Synthesis routes and methods I
Procedure details
Synthesis routes and methods II
Procedure details
Synthesis routes and methods III
Procedure details
Disclaimer and Information on In-Vitro Research Products
Please be aware that all articles and product information presented on BenchChem are intended solely for informational purposes. The products available for purchase on BenchChem are specifically designed for in-vitro studies, which are conducted outside of living organisms. In-vitro studies, derived from the Latin term "in glass," involve experiments performed in controlled laboratory settings using cells or tissues. It is important to note that these products are not categorized as medicines or drugs, and they have not received approval from the FDA for the prevention, treatment, or cure of any medical condition, ailment, or disease. We must emphasize that any form of bodily introduction of these products into humans or animals is strictly prohibited by law. It is essential to adhere to these guidelines to ensure compliance with legal and ethical standards in research and experimentation.