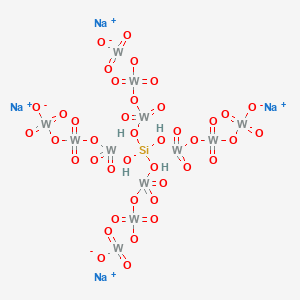
Sodium silicotungstate
- Click on QUICK INQUIRY to receive a quote from our team of experts.
- With the quality product at a COMPETITIVE price, you can focus more on your research.
Overview
Description
Sodium silicotungstate is a chemical compound that belongs to the class of heteropoly acids. It is known for its unique structure and properties, making it valuable in various scientific and industrial applications. The compound is often used as a catalyst and in negative staining techniques for electron microscopy due to its ability to provide high contrast and fine grain size .
Preparation Methods
Synthetic Routes and Reaction Conditions: Sodium silicotungstate can be synthesized by reacting sodium tungstate, silicon dioxide, and hydrochloric acid. The reaction typically involves dissolving sodium tungstate and silicon dioxide in water, followed by the addition of hydrochloric acid to precipitate the this compound .
Industrial Production Methods: In industrial settings, this compound is produced through a similar process but on a larger scale. The reactants are mixed in large reactors, and the resulting product is filtered, washed, and dried to obtain the final compound. The process is optimized to ensure high yield and purity of the product .
Chemical Reactions Analysis
Types of Reactions: Sodium silicotungstate undergoes various chemical reactions, including oxidation, reduction, and substitution reactions. It is particularly known for its catalytic properties in oxidation reactions .
Common Reagents and Conditions:
Oxidation Reactions: this compound acts as a catalyst in the oxidation of terpene alcohols using hydrogen peroxide as the oxidant.
Reduction Reactions: The compound can also participate in reduction reactions, although these are less common compared to oxidation reactions.
Major Products Formed: The major products formed from these reactions depend on the specific substrates used. For example, in the oxidation of terpene alcohols, the products can include epoxides, aldehydes, or ketones .
Scientific Research Applications
Sodium silicotungstate has a wide range of applications in scientific research:
Mechanism of Action
The mechanism by which sodium silicotungstate exerts its effects is primarily through its catalytic activity. The compound’s structure allows it to facilitate various chemical reactions by providing active sites for the reactants. In oxidation reactions, for example, this compound can activate hydrogen peroxide, enabling the transfer of oxygen atoms to the substrate . The molecular targets and pathways involved depend on the specific reaction and substrate being used.
Comparison with Similar Compounds
Silicotungstic Acid: Similar to sodium silicotungstate, silicotungstic acid is also a heteropoly acid with catalytic properties.
Phosphotungstic Acid: Another heteropoly acid, phosphotungstic acid, is used in similar applications but has different structural and catalytic properties.
Uniqueness: this compound is unique due to its ability to act as a heterogeneous catalyst, which allows for easier separation and recycling of the catalyst compared to homogeneous catalysts like silicotungstic acid. Additionally, its fine grain size and high contrast make it particularly valuable in electron microscopy .
Properties
IUPAC Name |
tetrasodium;dioxotungsteniooxy-(oxido(dioxo)tungstenio)oxy-dioxotungsten;silicic acid |
Source
|
---|---|---|
Source | PubChem | |
URL | https://pubchem.ncbi.nlm.nih.gov | |
Description | Data deposited in or computed by PubChem | |
InChI |
InChI=1S/4Na.H4O4Si.36O.12W/c;;;;1-5(2,3)4;;;;;;;;;;;;;;;;;;;;;;;;;;;;;;;;;;;;;;;;;;;;;;;;/h;;;;1-4H;;;;;;;;;;;;;;;;;;;;;;;;;;;;;;;;;;;;;;;;;;;;;;;;/q4*+1;;;;;;;;;;;;;;;;;;;;;;;;;;;;;;;;;;4*-1;;;;;;;;;;;; |
Source
|
Source | PubChem | |
URL | https://pubchem.ncbi.nlm.nih.gov | |
Description | Data deposited in or computed by PubChem | |
InChI Key |
RFKXKZJSLAFLCE-UHFFFAOYSA-N |
Source
|
Source | PubChem | |
URL | https://pubchem.ncbi.nlm.nih.gov | |
Description | Data deposited in or computed by PubChem | |
Canonical SMILES |
O[Si](O)(O)O.[O-][W](=O)(=O)O[W](=O)(=O)O[W](=O)=O.[O-][W](=O)(=O)O[W](=O)(=O)O[W](=O)=O.[O-][W](=O)(=O)O[W](=O)(=O)O[W](=O)=O.[O-][W](=O)(=O)O[W](=O)(=O)O[W](=O)=O.[Na+].[Na+].[Na+].[Na+] |
Source
|
Source | PubChem | |
URL | https://pubchem.ncbi.nlm.nih.gov | |
Description | Data deposited in or computed by PubChem | |
Molecular Formula |
H4Na4O40SiW12 |
Source
|
Source | PubChem | |
URL | https://pubchem.ncbi.nlm.nih.gov | |
Description | Data deposited in or computed by PubChem | |
Molecular Weight |
2970.1 g/mol |
Source
|
Source | PubChem | |
URL | https://pubchem.ncbi.nlm.nih.gov | |
Description | Data deposited in or computed by PubChem | |
Disclaimer and Information on In-Vitro Research Products
Please be aware that all articles and product information presented on BenchChem are intended solely for informational purposes. The products available for purchase on BenchChem are specifically designed for in-vitro studies, which are conducted outside of living organisms. In-vitro studies, derived from the Latin term "in glass," involve experiments performed in controlled laboratory settings using cells or tissues. It is important to note that these products are not categorized as medicines or drugs, and they have not received approval from the FDA for the prevention, treatment, or cure of any medical condition, ailment, or disease. We must emphasize that any form of bodily introduction of these products into humans or animals is strictly prohibited by law. It is essential to adhere to these guidelines to ensure compliance with legal and ethical standards in research and experimentation.