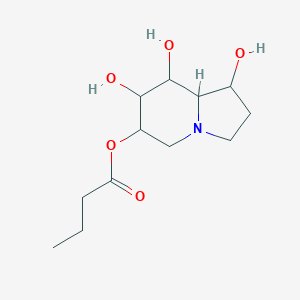
Celgosivir
Overview
Description
Celgosivir (6-O-butanoyl castanospermine) is an iminosugar prodrug of castanospermine, a natural alkaloid derived from the seeds of Castanospermum australe. It inhibits endoplasmic reticulum (ER) α-glucosidases I and II, enzymes critical for N-linked glycan processing of viral glycoproteins. By disrupting viral glycoprotein maturation, this compound impairs virion assembly and secretion, rendering viral particles non-infectious .
This compound was developed to overcome the poor oral bioavailability and gastrointestinal toxicity (e.g., osmotic diarrhea) associated with castanospermine. The addition of a lipophilic butanoyl side chain enhances intestinal absorption, resulting in 30–50-fold higher systemic exposure compared to castanospermine . Preclinical studies demonstrated broad-spectrum antiviral activity against flaviviruses (e.g., dengue, Zika), hepatitis C virus (HCV), bovine viral diarrhea virus (BVDV), and coronaviruses (e.g., SARS-CoV-2) .
Preparation Methods
Synthetic Route Development and Optimization
Core Synthesis via C-Acylation of Castanospermine
The foundational method for celgosivir synthesis involves the selective C-acylation of castanospermine, a natural alkaloid isolated from Castanospermum australe. The process, detailed in multiple preclinical and clinical studies, follows a four-step sequence:
-
Activation of Castanospermine : A suspension of castanospermine and bis(tributyltin) oxide (1:2 molar ratio) in anhydrous toluene is refluxed under argon for 3 hours to form a stannane intermediate .
-
Acylation with Butyryl Chloride : The reaction mixture is cooled to −17°C, followed by dropwise addition of butyryl chloride (1.8 molar excess) over 10 minutes. Stirring at room temperature for 2 hours facilitates esterification at the C6 hydroxyl group .
-
Quenching and Acidification : Absolute ethanol is added to hydrolyze residual tin byproducts, followed by 1.5M HCl/ethanol solution (2:1 molar ratio to butyryl chloride) to protonate the product. The mixture is stirred overnight at room temperature and chilled to 3°C to precipitate crude this compound .
-
Purification : The precipitate is filtered, washed with hexane, and recrystallized to achieve >99% purity by HPLC .
Key Reaction Parameters
Alternative Synthetic Strategies
While C-acylation remains the dominant industrial method, exploratory routes have been evaluated:
-
Enzymatic Acylation : Early attempts using lipases (e.g., Candida antarctica Lipase B) achieved partial regioselectivity but yielded <50% this compound due to competing acylations at C1 and C7 positions .
-
Solid-Phase Synthesis : Immobilized castanospermine on Wang resin showed promise in small-scale trials but suffered from low recovery rates (≤30%) and resin degradation .
Analytical and Process Validation
Structural Confirmation
Post-synthesis characterization employs multi-modal techniques:
-
Liquid Chromatography-Mass Spectrometry (LC/MS/MS) : Quantifies this compound and its metabolite castanospermine using a Waters Atlantis HILIC Silica column (23% 20 mM ammonium acetate pH 5.0, 77% acetonitrile) . Internal standards include N-dodecyl-deoxynojirimycin (this compound) and 6,7-dihydroxyswainsonine (castanospermine) .
-
Nuclear Magnetic Resonance (NMR) : H NMR (500 MHz, DO) confirms acylation at C6: δ 4.85 (d, J = 3.5 Hz, H-6), 2.35 (t, J = 7.4 Hz, CHCO), 1.55 (m, CHCHCH) .
Analytical Data Comparison
Parameter | This compound | Castanospermine |
---|---|---|
Retention Time (HPLC) | 7.2 min | 5.8 min |
Molecular Ion (m/z) | 260.1 [M+H] | 174.1 [M+H] |
Purity Threshold | >99% | >98% |
Scalability and GMP Compliance
Large-scale production (e.g., Dalton Pharma Services) adheres to Good Manufacturing Practice (GMP) guidelines:
-
Capsule Formulation : this compound is encapsulated in 100 mg doses using pregelatinized maize starch as an excipient, with blister packs ensuring stability under 25°C/60% RH .
-
Batch Consistency : Inter-batch variability for C6 acylation is <2% (n=12), validated by LC/MS/MS .
Process-Related Impurities and Mitigation
Identified Impurities
-
Castanospermine (0.5–1.2%) : Incomplete acylation or ester hydrolysis during storage .
-
Tin Residues (≤10 ppm) : Traces from bis(tributyltin) oxide, removed via ethanol/HCl washes .
Stability Optimization
Accelerated stability studies (40°C/75% RH, 6 months) show:
Chemical Reactions Analysis
Types of Reactions
Celgosivir undergoes various chemical reactions, including:
Oxidation: The hydroxyl groups can be oxidized to form ketones or aldehydes.
Reduction: The compound can be reduced to form alcohols or other reduced derivatives.
Substitution: The ester group can undergo nucleophilic substitution reactions, leading to the formation of different esters or amides.
Common Reagents and Conditions
Oxidation: Common oxidizing agents include potassium permanganate (KMnO4) and chromium trioxide (CrO3).
Reduction: Reducing agents such as lithium aluminum hydride (LiAlH4) or sodium borohydride (NaBH4) are typically used.
Substitution: Nucleophiles like ammonia (NH3) or primary amines (RNH2) can be used for substitution reactions.
Major Products Formed
The major products formed from these reactions depend on the specific conditions and reagents used. For example, oxidation may yield ketones or aldehydes, while reduction can produce alcohols. Substitution reactions can result in the formation of various esters or amides.
Scientific Research Applications
Celgosivir has several scientific research applications, including:
Chemistry: Used as a building block for the synthesis of more complex molecules.
Biology: Studied for its potential biological activity and interactions with enzymes or receptors.
Medicine: Investigated for its potential therapeutic effects, such as antiviral or anticancer properties.
Industry: Utilized in the production of specialty chemicals and materials.
Mechanism of Action
The mechanism of action of Celgosivir involves its interaction with specific molecular targets, such as enzymes or receptors. The hydroxyl groups and ester functionality allow the compound to form hydrogen bonds and other interactions with biological molecules, potentially modulating their activity. For example, it may inhibit certain enzymes by binding to their active sites, thereby affecting metabolic pathways.
Comparison with Similar Compounds
Celgosivir belongs to the iminosugar class of α-glucosidase inhibitors. Key comparisons with structurally and mechanistically related compounds are outlined below:
Castanospermine
- Mechanism: Both inhibit ER α-glucosidases I/II, but this compound acts as a prodrug, metabolized into castanospermine .
- Potency : this compound exhibits 7–100-fold greater antiviral efficacy in vitro against dengue virus (DENV) and BVDV due to enhanced cellular uptake .
- Pharmacokinetics: this compound achieves 30-fold higher plasma concentrations of castanospermine than direct castanospermine administration .
- Toxicity: Castanospermine causes dose-limiting diarrhea by inhibiting intestinal disaccharidases, whereas this compound minimizes this side effect .
UV-4B
- Structure: UV-4B is a deoxy-nojirimycin (DNJ) derivative with a hydroxylated alkyl side chain.
- Activity : Both compounds inhibit ER α-glucosidases, but UV-4B shows superior in vitro potency against DENV (EC₅₀ = 0.5 μM vs. This compound’s EC₅₀ = 5 μM) .
- Clinical Trials : UV-4B trials were terminated due to business constraints, while this compound advanced to Phase II for dengue and HCV .
Australine
- Source: Derived from Castanospermum australe, australine is a tetrahydroxypyrrolizidine alkaloid.
- Specificity : Australine selectively inhibits α1–4 and α1–6 exo-glucosidases but has weak activity against ER α-glucosidase II compared to this compound .
- Antiviral Efficacy : this compound reduces SARS-CoV-2 replication and spike protein levels at 10–100 μM, whereas australine requires higher concentrations for comparable effects .
Miglustat
- Approved Use : Miglustat, a DNJ derivative, is used for Gaucher’s disease and Niemann-Pick type C.
- Mechanism: Unlike this compound, miglustat targets glucosylceramide synthase, impacting glycolipid biosynthesis.
Data Tables
Table 1: In Vitro Antiviral Activity of this compound vs. Comparators
Table 2: Clinical Trial Outcomes
Key Research Findings
- Dengue Clinical Trials : The CELADEN Phase Ib trial (NCT01619969) found this compound safe but ineffective at reducing viremia or fever burden. However, secondary endpoints suggested reduced thrombocytopenia and TNFα levels in patients with secondary dengue infections .
- HCV Synergy: this compound combined with PegIFN-α/ribavirin reduced HCV RNA by 1.5–2.0 log₁₀ in non-responders, supporting host-targeted combination strategies .
- SARS-CoV-2 Inhibition : this compound reduced viral replication by 90% at 100 μM in Vero E6 cells, but clinical relevance remains unexplored .
Q & A
Basic Research Questions
Q. What is the established mechanism of action of Celgosivir against flaviviruses, and how is this validated experimentally?
this compound acts as an α-glucosidase I inhibitor, disrupting viral glycoprotein maturation by preventing the trimming of N-linked oligosaccharides. This mechanism is validated using in vitro assays measuring viral replication inhibition (e.g., plaque reduction in BVDV with IC₅₀ = 1.27 μM) and glycoprotein accumulation in HIV-infected cells (IC₅₀ = 254 μM) . Methodologically, researchers employ immunoblotting to detect immature glycoproteins and viral titer quantification via plaque assays to confirm efficacy .
Q. Which experimental models are most relevant for assessing this compound’s antiviral activity, and what endpoints are critical?
Preclinical models include:
- Cell-based systems : DENV-2 (EC₅₀ = 0.2 μM), BVDV (IC₅₀ = 16–47 μM), and Zika virus (PRVABC59 strain) .
- Animal models : Lethal mouse models for dengue (DENV-2) to evaluate dose-dependent survival rates and viremia reduction . Key endpoints include viral RNA load (qRT-PCR), plaque-forming units (PFU), and cell viability assays (e.g., MTT for cytotoxicity) .
Q. How do researchers distinguish between this compound’s direct antiviral effects and host-directed activity in vitro?
Studies use time-of-addition assays to determine the stage of viral replication inhibited (e.g., pre- vs. post-entry). For example, this compound’s inhibition of α-glucosidase I (a host enzyme) is confirmed by comparing its effects with viral protease inhibitors. Co-treatment with castanospermine (the active metabolite) further validates target specificity .
Advanced Research Questions
Q. What methodological approaches resolve contradictions between this compound’s in vitro potency and limited clinical efficacy in dengue trials?
The CELADEN trial (NCT01619969) highlighted discordance between in vitro EC₅₀ values (0.2 μM for DENV-2) and clinical outcomes. Researchers address this via:
- Pharmacokinetic (PK) profiling : Measuring plasma concentrations (e.g., 400 mg loading dose vs. 200 mg bid) to assess drug exposure relative to the in vitro effective range .
- Viral sequencing : Next-generation sequencing (NGS) identifies potential resistance mutations in NS1 or envelope proteins during treatment .
- Immunological correlates : Linking platelet counts, NS1 clearance rates, and hematocrit trends to antiviral activity .
Q. How can researchers optimize combination therapy strategies with this compound for enhanced antiviral synergy?
Synergy is tested using Loewe additivity or Bliss independence models in in vitro systems. For HCV, this compound combined with interferon-α2b and ribavirin showed enhanced viral reduction. Methodological steps include:
- Dose-matrix designs to identify synergistic ratios.
- Mechanistic complementarity analysis (e.g., pairing host-targeted this compound with viral polymerase inhibitors) .
Q. What analytical techniques are critical for characterizing this compound’s pharmacokinetic-pharmacodynamic (PK/PD) relationships in vivo?
- Liquid chromatography-tandem mass spectrometry (LC-MS/MS) : Quantifies plasma levels of this compound and castanospermine .
- Compartmental modeling : Links drug exposure to viral load reduction or hematological endpoints (e.g., platelet recovery) .
- Population PK analysis : Accounts for inter-patient variability in drug metabolism and clearance .
Q. How should researchers design experiments to evaluate this compound’s potential against emerging arboviruses (e.g., Zika)?
- Plaque reduction neutralization tests (PRNT) in Vero cells infected with Zika virus (e.g., PRVABC59 strain) to measure EC₅₀.
- Time-course assays : Compare viral RNA synthesis (RT-qPCR) and virion production (plaque assay) at 48 vs. 72 hours post-treatment, as done in Zika studies .
- In vivo efficacy : Use immunocompromised mice (e.g., AG129) to model Zika pathogenesis and assess survival, neuroinvasion, and viral burden in organs .
Q. Data Contradiction Analysis
Q. Why does this compound show variable IC₅₀ values across flaviviruses (e.g., DENV-2 EC₅₀ = 0.2 μM vs. BVDV IC₅₀ = 1.27 μM)?
Variability arises from:
- Viral dependency on α-glucosidase I : DENV’s envelope glycoproteins are more sensitive to improper glycosylation than BVDV’s.
- Cell-type differences : Antiviral activity in Huh-7 (HCV) vs. Vero (DENV/Zika) cells may reflect divergent host enzyme expression .
Q. How can researchers address the discrepancy between this compound’s preclinical success and clinical trial outcomes?
- Post hoc analysis : Stratify clinical data by viral serotype, immune status, or PK parameters to identify responsive subpopulations .
- Improved biomarkers : Use NS1 antigenemia or interferometric imaging to quantify drug-induced glycoprotein defects in patient samples .
Q. Experimental Design Considerations
Q. What controls are essential in this compound dose-response studies to ensure robustness?
- Vehicle controls : Account for solvent effects (e.g., DMSO cytotoxicity).
- Positive controls : Use established antivirals (e.g., ribavirin for DENV) to benchmark efficacy.
- Cytotoxicity controls : Parallel cell viability assays (e.g., MTT) to confirm antiviral effects are not due to cell death .
Q. How should researchers standardize assays for quantifying α-glucosidase I inhibition?
Properties
Molecular Formula |
C12H21NO5 |
---|---|
Molecular Weight |
259.30 g/mol |
IUPAC Name |
(1,7,8-trihydroxy-1,2,3,5,6,7,8,8a-octahydroindolizin-6-yl) butanoate |
InChI |
InChI=1S/C12H21NO5/c1-2-3-9(15)18-8-6-13-5-4-7(14)10(13)12(17)11(8)16/h7-8,10-12,14,16-17H,2-6H2,1H3 |
InChI Key |
HTJGLYIJVSDQAE-UHFFFAOYSA-N |
Canonical SMILES |
CCCC(=O)OC1CN2CCC(C2C(C1O)O)O |
Origin of Product |
United States |
Synthesis routes and methods
Procedure details
Disclaimer and Information on In-Vitro Research Products
Please be aware that all articles and product information presented on BenchChem are intended solely for informational purposes. The products available for purchase on BenchChem are specifically designed for in-vitro studies, which are conducted outside of living organisms. In-vitro studies, derived from the Latin term "in glass," involve experiments performed in controlled laboratory settings using cells or tissues. It is important to note that these products are not categorized as medicines or drugs, and they have not received approval from the FDA for the prevention, treatment, or cure of any medical condition, ailment, or disease. We must emphasize that any form of bodily introduction of these products into humans or animals is strictly prohibited by law. It is essential to adhere to these guidelines to ensure compliance with legal and ethical standards in research and experimentation.