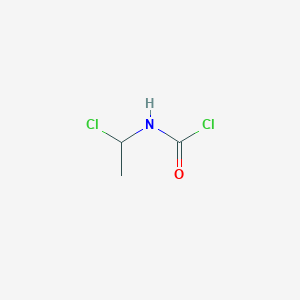
(1-Chloroethyl)carbamyl chloride
- Click on QUICK INQUIRY to receive a quote from our team of experts.
- With the quality product at a COMPETITIVE price, you can focus more on your research.
Overview
Description
(1-Chloroethyl)carbamyl chloride is a carbamyl chloride derivative characterized by a chloroethyl group (-CH₂CH₂Cl) attached to the carbamoyl moiety (-NCOCl). Carbamyl chlorides are reactive intermediates widely used in organic synthesis, particularly for introducing carbamate groups into target molecules . For instance, carbamyl chloride derivatives have been employed to synthesize antitumor agents and herbicides, highlighting their versatility in pharmaceutical and agrochemical research .
Scientific Research Applications
Cancer Research
One of the most prominent applications of (1-chloroethyl)carbamyl chloride is in cancer research. The compound has been shown to interact with DNA, causing modifications that can lead to cell cycle arrest and apoptosis in cancer cells. Its mechanism involves:
- DNA Cross-linking : The compound can induce cross-links between DNA strands, inhibiting replication and transcription, ultimately resulting in cell death.
- Inhibition of DNA Repair Enzymes : By targeting enzymes responsible for DNA repair, this compound exacerbates DNA damage, enhancing its cytotoxic effects on tumor cells.
Synthesis of Therapeutic Agents
This compound serves as a precursor in the synthesis of various therapeutic agents. It can be utilized to create derivatives with potential biological activity, including:
- Anticholinesterase Activity : Reacting with eseridine leads to compounds that exhibit anticholinesterase properties, useful in treating conditions like myasthenia gravis.
- Antimycobacterial Compounds : The synthesis of phenylureas from this compound has been linked to enhanced activity against mycobacteria .
Development of Herbicides and Pesticides
The compound's reactivity also extends to agricultural applications. Its derivatives are explored as herbicides and pesticides due to their ability to target specific biological pathways in plants and pests .
Case Study 1: Cytotoxicity in Cancer Cells
A study investigated the effects of this compound on various cancer cell lines. Results indicated that treatment led to significant apoptosis and reduced cell viability, correlating with increased levels of DNA damage markers. This highlights its potential as a chemotherapeutic agent.
Case Study 2: Synthesis Pathways
Research on synthetic pathways revealed that this compound could be synthesized efficiently through chlorination processes involving ethyl carbamyl chloride. This method offers a high yield and purity, making it suitable for large-scale production for research purposes .
Chemical Reactions Analysis
Nucleophilic Substitution Reactions
The compound undergoes nucleophilic displacement at both the carbamoyl chloride (-COCl) and chloroethyl (-CH₂Cl) groups:
a. Reaction with Amines
-
Primary/secondary amines attack the carbonyl carbon, forming substituted ureas (Table 1):
(1-Chloroethyl)carbamyl chloride+R-NH2→R-NH-CO-NH-(CH2Cl)+HCl
b. Hydrolysis
-
Controlled hydrolysis produces carbamic acid derivatives:
C3H5Cl2NO+H2O→C2H5Cl-NH-COOH+HCl
Elimination Reactions
Thermal or base-induced elimination generates reactive intermediates:
a. Isocyanate Formation
-
Pyrolysis at 90–120°C yields 1-chloroethyl isocyanate:
C3H5Cl2NOΔCl-CH2-CH2-NCO+HCl
b. β-Elimination with Bases
-
Strong bases (e.g., NaOH) induce dehydrohalogenation:
C3H5Cl2NO+NaOH→CH2=CH-NHCOCl+NaCl+H2O
Solvolysis Mechanisms
Kinetic studies reveal solvent-dependent pathways (Table 2):
Solvent System | Mechanism | k (s−1) | ΔS‡ (cal·mol⁻¹·K⁻¹) | Reference |
---|---|---|---|---|
89.1% Acetone–H₂O | Sₙ1 | 8.4×10−2 | +5.6 | |
Pure H₂O | Sₙ2 | 2.1×10−3 | -12.4 |
-
Sₙ1 Dominance in polar aprotic solvents due to carbocation stabilization.
-
Sₙ2 Preference in aqueous media with nucleophilic water participation.
Side Reactions and Byproducts
-
Dimerization : Prolonged UV exposure during synthesis forms bis(1-chloroethyl) carbonate (yield: 8–12%) .
-
Thiouronium Formation : Competing pathway with thioureas under basic conditions .
Table 1: Representative Substitution Reactions
Table 2: Solvolysis Kinetics
Substrate | Solvent | Ea (kcal/mol) | log A (s⁻¹) | Reference |
---|---|---|---|---|
(1-Chloroethyl)carbamyl Cl | 89.1% Acetone | 21.0 | 9.8 | |
Dimethylcarbamoyl Cl | Pure H₂O | 20.6 | 8.2 |
Radical-Mediated Pathways
Q & A
Basic Research Questions
Q. What are the standard synthetic pathways for (1-chloroethyl)carbamyl chloride, and how can reaction conditions be optimized to improve yield?
- Methodological Answer : The synthesis typically involves chlorination of carbamyl precursors using reagents like thionyl chloride or phosgene derivatives. Optimization requires controlling stoichiometry, reaction temperature (e.g., maintaining ≤40°C to minimize side reactions), and inert atmosphere conditions to prevent hydrolysis. Reaction progress can be monitored via FTIR for carbonyl chloride (C=O stretch at ~1800 cm⁻¹) and TLC to track intermediates. Post-synthesis purification via fractional distillation or column chromatography is critical for isolating high-purity product .
Q. Which analytical techniques are most reliable for characterizing this compound, and how should data interpretation be validated?
- Methodological Answer :
- NMR Spectroscopy : Use 1H and 13C NMR to confirm the chloroethyl group (δ ~4.5 ppm for CH₂Cl) and carbamyl carbonyl (δ ~155-160 ppm).
- Mass Spectrometry : High-resolution MS (HRMS) identifies molecular ion peaks ([M+Na]⁺ or [M+H]⁺) and fragmentation patterns.
- Elemental Analysis : Validate Cl content via combustion analysis.
- Cross-validate results with independent methods (e.g., comparing NMR with IR) to ensure consistency. Maintain calibration standards and replicate measurements to address instrumental variability .
Q. What safety protocols are essential when handling this compound in laboratory settings?
- Methodological Answer :
- Personal Protective Equipment (PPE) : Wear nitrile gloves, chemical-resistant aprons, and full-face shields.
- Ventilation : Use fume hoods to prevent inhalation of volatile chlorides.
- Spill Management : Neutralize spills with sodium bicarbonate or specialized absorbents.
- First Aid : Immediate rinsing with water for skin/eye contact and medical consultation for inhalation exposure. Reference Safety Data Sheets (SDS) for compound-specific hazards .
Q. How can researchers assess the stability of this compound under varying storage conditions?
- Methodological Answer : Conduct accelerated stability studies by storing samples at different temperatures (-20°C, 4°C, 25°C) and humidity levels. Monitor degradation via periodic HPLC analysis to quantify hydrolyzed byproducts (e.g., carbamic acid). Use Arrhenius kinetics to extrapolate shelf-life under standard conditions. Document deviations from ideal storage parameters .
Advanced Research Questions
Q. What mechanistic insights explain the reactivity of this compound in nucleophilic acyl substitution reactions?
- Methodological Answer : The electron-withdrawing chloroethyl group enhances electrophilicity at the carbonyl carbon, facilitating nucleophilic attack. Kinetic studies (e.g., UV-Vis monitoring of reaction rates with amines or alcohols) can elucidate steric and electronic effects. Computational modeling (DFT) further predicts transition states and regioselectivity, validated by experimental 13C NMR shifts .
Q. How should researchers address contradictions in reported toxicity data for this compound?
- Methodological Answer :
- Data Triangulation : Compare carcinogenicity studies (e.g., rodent models vs. in vitro assays) while noting dose-response variability.
- Confounding Factors : Control for impurities (e.g., residual phosgene) in toxicity assays.
- Statistical Analysis : Apply ANOVA or Bayesian models to assess significance of conflicting results.
- Literature Review : Prioritize studies adhering to OECD/ICH guidelines for reproducibility .
Q. What experimental strategies can isolate the effects of this compound’s stereochemistry on its bioactivity?
- Methodological Answer : Synthesize enantiomers via chiral auxiliaries or asymmetric catalysis. Use chiral HPLC or polarimetry to confirm enantiomeric purity. Compare biological activity (e.g., enzyme inhibition assays) between enantiomers, and employ X-ray crystallography to resolve stereochemical interactions with target proteins .
Q. How can computational modeling predict the environmental degradation pathways of this compound?
- Methodological Answer : Use QSAR (Quantitative Structure-Activity Relationship) models to estimate hydrolysis rates in aquatic environments. Molecular dynamics simulations can simulate interactions with water and soil components. Validate predictions with GC-MS analysis of degradation products (e.g., CO₂, ethylamine derivatives) in controlled environmental chambers .
Q. What methodologies are recommended for studying structure-activity relationships (SAR) of this compound derivatives?
- Methodological Answer :
- Derivatization : Synthesize analogs with modified chloroethyl or carbamyl groups.
- Bioassays : Test analogs in cell-based cytotoxicity or receptor-binding assays.
- Multivariate Analysis : Apply PCA (Principal Component Analysis) to correlate structural descriptors (e.g., Hammett σ values) with bioactivity data.
- Crystallography : Resolve 3D structures of protein-ligand complexes to identify key binding motifs .
Q. Data Presentation Guidelines
- Tables : Include spectral data (e.g., NMR shifts, IR peaks) with error margins.
- Graphs : Plot kinetic data with error bars and regression lines (R² ≥0.98 for reliability).
- Reproducibility : Document experimental assumptions, deviations, and raw data in appendices .
Comparison with Similar Compounds
Chloroacetyl Chloride (ClCH₂COCl)
Chloroacetyl chloride shares structural similarities with (1-Chloroethyl)carbamyl chloride, as both contain a chlorine atom adjacent to an electrophilic carbonyl group. However, chloroacetyl chloride lacks the carbamoyl nitrogen, making it more reactive toward nucleophiles like alcohols and amines. Key distinctions include:
- Applications : Chloroacetyl chloride is primarily used in synthesizing herbicides and pharmaceuticals, whereas carbamyl chlorides are favored for carbamate formation .
- Hazards : Chloroacetyl chloride is corrosive and a potent lachrymator, requiring strict handling precautions (UN 1752) .
Dimethylcarbamyl Chloride ((CH₃)₂NCOCl)
Dimethylcarbamyl chloride, a tertiary carbamyl chloride, exhibits reduced reactivity compared to primary analogs due to steric hindrance from methyl groups. Key differences:
- Synthetic Utility : It is used to prepare urea derivatives (e.g., herbicidal compounds) via reactions with amines .
Carbamyl Chloride (H₂NCOCl)
The parent compound, carbamyl chloride, is highly unstable and typically generated in situ using triphosgene. It serves as a precursor for antitumor caged xanthones, with yields of 50–70% in such reactions . Unlike this compound, it lacks the chloroethyl group, which may alter solubility and stability.
Data Table: Comparative Analysis of Carbamyl Chloride Derivatives
Properties
CAS No. |
70008-75-2 |
---|---|
Molecular Formula |
C3H5Cl2NO |
Molecular Weight |
141.98 g/mol |
IUPAC Name |
N-(1-chloroethyl)carbamoyl chloride |
InChI |
InChI=1S/C3H5Cl2NO/c1-2(4)6-3(5)7/h2H,1H3,(H,6,7) |
InChI Key |
LVQSPZHLYFKOAS-UHFFFAOYSA-N |
Canonical SMILES |
CC(NC(=O)Cl)Cl |
Origin of Product |
United States |
Disclaimer and Information on In-Vitro Research Products
Please be aware that all articles and product information presented on BenchChem are intended solely for informational purposes. The products available for purchase on BenchChem are specifically designed for in-vitro studies, which are conducted outside of living organisms. In-vitro studies, derived from the Latin term "in glass," involve experiments performed in controlled laboratory settings using cells or tissues. It is important to note that these products are not categorized as medicines or drugs, and they have not received approval from the FDA for the prevention, treatment, or cure of any medical condition, ailment, or disease. We must emphasize that any form of bodily introduction of these products into humans or animals is strictly prohibited by law. It is essential to adhere to these guidelines to ensure compliance with legal and ethical standards in research and experimentation.