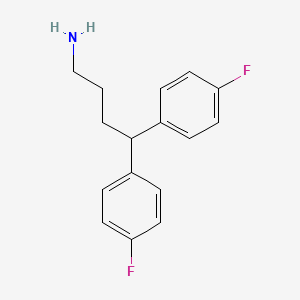
4,4-Bis(4-fluorophenyl)butan-1-amine
- Click on QUICK INQUIRY to receive a quote from our team of experts.
- With the quality product at a COMPETITIVE price, you can focus more on your research.
Description
4,4-Bis(4-fluorophenyl)butan-1-amine is a useful research compound. Its molecular formula is C16H17F2N and its molecular weight is 261.31 g/mol. The purity is usually 95%.
BenchChem offers high-quality this compound suitable for many research applications. Different packaging options are available to accommodate customers' requirements. Please inquire for more information about this compound including the price, delivery time, and more detailed information at info@benchchem.com.
Scientific Research Applications
Chemistry
4,4-Bis(4-fluorophenyl)butan-1-amine serves as a crucial building block in organic synthesis. Its unique structure allows it to participate in various chemical reactions, including oxidation, reduction, and substitution. These reactions enable the formation of more complex molecules, making it valuable in the development of new materials and chemicals .
Biology
Research has indicated that this compound exhibits potential biological activities. It is being investigated for its interactions with biomolecules and its effects on various biological pathways. Preliminary studies suggest that it may modulate neurotransmitter receptors, which could have implications for treating neurological disorders .
Medicine
The compound is being explored for its therapeutic properties. It has shown promise as a precursor in drug development, particularly in creating new pharmacological agents targeting specific diseases. For instance, derivatives of this compound have been studied for their anticancer activity against various cell lines .
Case Study: Anticancer Activity
A study examining analogs of this compound demonstrated significant cytotoxic effects against multiple cancer cell lines. The introduction of electron-donating groups enhanced the potency of these compounds, highlighting the importance of structure-activity relationships (SAR) in drug design .
Compound | Cell Line | IC50 (µM) |
---|---|---|
Compound A | HCT-116 (Colon Cancer) | 92.4 |
Compound B | MCF-7 (Breast Cancer) | 0.11 |
Compound C | A375 (Melanoma) | 2.09 |
Industry
In industrial applications, this compound is utilized in producing specialty chemicals and materials. Its properties make it suitable for formulating products with specific functionalities required in various sectors .
Properties
Molecular Formula |
C16H17F2N |
---|---|
Molecular Weight |
261.31 g/mol |
IUPAC Name |
4,4-bis(4-fluorophenyl)butan-1-amine |
InChI |
InChI=1S/C16H17F2N/c17-14-7-3-12(4-8-14)16(2-1-11-19)13-5-9-15(18)10-6-13/h3-10,16H,1-2,11,19H2 |
InChI Key |
WCBVVQJSZZESAF-UHFFFAOYSA-N |
Canonical SMILES |
C1=CC(=CC=C1C(CCCN)C2=CC=C(C=C2)F)F |
Origin of Product |
United States |
Disclaimer and Information on In-Vitro Research Products
Please be aware that all articles and product information presented on BenchChem are intended solely for informational purposes. The products available for purchase on BenchChem are specifically designed for in-vitro studies, which are conducted outside of living organisms. In-vitro studies, derived from the Latin term "in glass," involve experiments performed in controlled laboratory settings using cells or tissues. It is important to note that these products are not categorized as medicines or drugs, and they have not received approval from the FDA for the prevention, treatment, or cure of any medical condition, ailment, or disease. We must emphasize that any form of bodily introduction of these products into humans or animals is strictly prohibited by law. It is essential to adhere to these guidelines to ensure compliance with legal and ethical standards in research and experimentation.