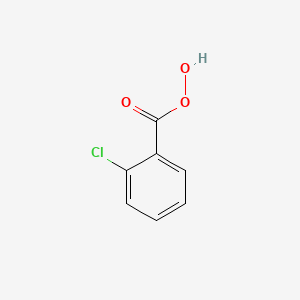
2-Chlorobenzenecarboperoxoic acid
- Click on QUICK INQUIRY to receive a quote from our team of experts.
- With the quality product at a COMPETITIVE price, you can focus more on your research.
Overview
Description
2-Chlorobenzenecarboperoxoic acid is an organic compound characterized by a benzene ring substituted with a chlorine atom at the ortho position and a peroxoic acid (-COOOH) functional group. This structure confers unique oxidative and reactive properties, making it relevant in synthetic chemistry, particularly in epoxidation and oxidation reactions. Its stability and reactivity are influenced by the electron-withdrawing chlorine substituent and the labile peroxo bond, which differentiates it from simpler carboxylic acid derivatives like 2-chlorobenzoic acid .
Q & A
Basic Research Questions
Q. What spectroscopic techniques are most effective for characterizing 2-Chlorobenzenecarboperoxoic acid, and what key peaks should be identified?
- Methodological Answer :
- Infrared (IR) Spectroscopy : The O-O stretch of the peroxy group appears at ~800–900 cm⁻¹. The carboxylic acid (C=O) stretch is observed near 1700 cm⁻¹.
- NMR Spectroscopy :
- ¹H NMR : Chlorine’s electron-withdrawing effect deshields adjacent aromatic protons, causing distinct splitting patterns. For example, in similar chloro-benzoic acids, meta-protons to chlorine show shifts near δ 7.5–8.0 ppm .
- ¹³C NMR : The carboxylic carbon appears at ~170 ppm, while the peroxy carbon is near 90–100 ppm.
- Mass Spectrometry (MS) : Look for the molecular ion peak ([M+H]⁺) corresponding to the molecular formula. Fragmentation patterns should confirm the peroxy and chloro substituents.
Q. How can researchers ensure the stability of this compound during storage and experimental use?
- Methodological Answer :
- Store in airtight, light-resistant containers at –20°C to slow decomposition.
- Use stabilizers like hydroquinone or BHT to inhibit radical-mediated degradation.
- Monitor purity via HPLC (C18 column, mobile phase: acetonitrile/water with 0.1% TFA) or TLC (silica gel, Rf ~0.5 in ethyl acetate/hexane).
- Regularly calibrate analytical instruments to detect early signs of decomposition .
Advanced Research Questions
Q. How can computational chemistry predict the reaction pathways of this compound in complex organic transformations?
- Methodological Answer :
- Density Functional Theory (DFT) : Model transition states for peracid-mediated oxidations (e.g., epoxidation of alkenes). Compare activation energies of competing pathways.
- Molecular Dynamics (MD) : Simulate solvent effects (e.g., polarity of dichloromethane vs. water) on reaction kinetics.
- Validate predictions with experimental kinetic data (e.g., rate constants measured via UV-Vis spectroscopy).
- Patent filings for analogous peracids suggest applications in asymmetric catalysis, which can guide hypothesis generation .
Q. What strategies resolve contradictions in reported oxidative efficiencies of this compound across solvent systems?
- Methodological Answer :
- Controlled Experiments : Systematically vary solvent polarity (e.g., using Kamlet-Taft parameters) and measure reaction yields.
- Statistical Analysis : Apply multivariate regression to isolate solvent effects from other variables (temperature, concentration).
- Meta-Analysis : Aggregate literature data to identify trends, excluding studies with inconsistent methodologies (e.g., non-standardized purity checks).
- Cross-reference findings with computational solvent models to reconcile discrepancies .
Q. How does the chloro substituent influence the electronic properties and catalytic activity of this compound compared to non-halogenated analogs?
- Methodological Answer :
- Hammett Constants : Use σₚ values to quantify the electron-withdrawing effect of chlorine. Compare reaction rates (e.g., epoxidation) with meta- and para-substituted analogs.
- Cyclic Voltammetry : Measure redox potentials to assess the peracid’s oxidizing strength. Chlorine typically lowers the LUMO energy, enhancing electrophilicity.
- X-ray Crystallography : Resolve crystal structures to correlate substituent positioning with reactivity trends. PubChem data for related compounds provide benchmarks for structural comparisons .
Q. Data Contradiction and Validation
Q. What methodologies are recommended for analyzing conflicting stability data of this compound in academic studies?
- Methodological Answer :
- Standardized Protocols : Replicate studies under controlled conditions (pH 7.0 buffer, 25°C) to isolate environmental variables.
- Accelerated Stability Testing : Use elevated temperatures (40–60°C) to extrapolate degradation kinetics via the Arrhenius equation.
- Collaborative Trials : Share samples between labs to verify reproducibility. Discrepancies may arise from impurities or analytical method sensitivity .
Comparison with Similar Compounds
Comparison with Structurally Similar Compounds
2-Chlorobenzoic Acid (CASRN 118-91-2)
- Structural Similarity : Both compounds share a benzene ring with a chlorine substituent at the ortho position. However, 2-chlorobenzenecarboperoxoic acid replaces the carboxylic acid (-COOH) group with a peroxoic acid (-COOOH) moiety.
- Toxicity: The U.S. EPA notes that 2-chlorobenzoic acid lacks sufficient repeated-dose toxicity data, complicating direct comparisons with its peroxoic analog .
Other Chlorinated Benzenecarboxylic Acids
Compounds like 2,4-dichlorobenzoic acid and 3-chlorobenzoic acid exhibit similar halogenated aromatic frameworks but differ in substitution patterns. For example:
- Stability : The peroxoic acid derivative is less stable due to the O-O bond’s susceptibility to thermal or photolytic cleavage.
- Applications : Chlorinated benzoic acids are often intermediates in agrochemical synthesis, whereas the peroxoic variant is specialized for oxidation reactions .
Non-Chlorinated Peroxoic Acids
- Example : Meta-chloroperoxybenzoic acid (mCPBA), a widely used oxidizing agent.
- Comparison : The absence of an ortho-chlorine substituent in mCPBA reduces steric hindrance, enhancing its reactivity in certain stereoselective reactions compared to this compound.
Data Tables
Table 1: Structural and Functional Comparison
Compound | Functional Groups | Key Reactivity | Stability Concerns |
---|---|---|---|
This compound | -Cl, -COOOH | Epoxidation, oxidation of sulfides | Thermal decomposition |
2-Chlorobenzoic acid | -Cl, -COOH | Acid catalysis, salt formation | High thermal stability |
mCPBA | -Cl (meta), -COOOH | Stereoselective oxidations | Moderate stability in solution |
Table 2: Toxicity and Regulatory Data
Compound | Toxicity Data Availability | Regulatory Notes |
---|---|---|
This compound | Limited | No EPA screening values; inferred hazards |
2-Chlorobenzoic acid | Partial (acute only) | EPA provisional toxicity values pending |
mCPBA | Well-documented | Classified as irritant; OSHA-regulated |
Key Research Findings
- Reactivity : The ortho-chlorine in this compound sterically hinders electrophilic attack, reducing reaction rates compared to para-substituted analogs .
- Toxicity Gaps : The U.S. EPA identified eight structurally similar compounds to 2-chlorobenzoic acid (similarity >70%) but found insufficient data to establish read-across toxicity profiles for peroxoic derivatives .
- Synthetic Utility : Despite stability challenges, the compound’s strong oxidizing power makes it valuable in niche applications, such as synthesizing epoxides inaccessible via other peroxoic acids.
Preparation Methods
Synthesis via Acid Chloride Peroxidation
The most widely documented method for preparing mCPBA involves the reaction of 3-chlorobenzoyl chloride with hydrogen peroxide under acidic conditions. This two-step process begins with the synthesis of 3-chlorobenzoic acid, followed by its conversion to the corresponding peracid.
Preparation of 3-Chlorobenzoic Acid
3-Chlorobenzoic acid serves as the precursor for mCPBA synthesis. Industrial-scale production typically employs the oxidation of 3-chlorotoluene using potassium permanganate (KMnO₄) in aqueous acidic media . Alternatively, hydrolysis of 3-chlorobenzonitrile with concentrated hydrochloric acid at elevated temperatures (100–110°C) achieves near-quantitative yields (98%) .
Peroxidation of 3-Chlorobenzoyl Chloride
The acid chloride derivative of 3-chlorobenzoic acid reacts with hydrogen peroxide in the presence of sulfuric acid to yield mCPBA. A representative procedure involves:
-
Dissolving 3-chlorobenzoyl chloride (52.5 g) in dioxane.
-
Gradual addition of 30% hydrogen peroxide (90 mL) and sulfuric acid at 15–25°C.
-
Isolation of the product via vacuum distillation, achieving a 92% yield .
This method prioritizes reaction temperature control to minimize undesired side reactions, such as over-oxidation or decomposition. The use of dioxane as a solvent enhances miscibility between the organic acid chloride and aqueous peroxide phases.
Direct Oxidation of 3-Chlorobenzoic Acid
Recent advancements have enabled the direct conversion of 3-chlorobenzoic acid to mCPBA using phase-transfer catalysis (PTC). This single-step approach offers operational simplicity and reduced environmental impact compared to traditional methods.
Phase-Transfer Catalyzed Oxidation
A optimized protocol involves:
-
Dissolving 3-chlorobenzoic acid (3.91 g) in chloroform.
-
Adding acidic potassium permanganate (pH = 1) and benzyl triphenylphosphine chloride (0.97 g) as the PTC.
-
Maintaining the reaction at 15°C for 8 hours, followed by extraction and drying .
This method achieves 85–90% conversion efficiency, with the PTC facilitating interfacial electron transfer between the solid permanganate and organic phases. The mild reaction conditions prevent thermal degradation of the peroxide product.
Comparative Analysis of Synthetic Methods
The table below contrasts key parameters of the two primary preparation routes:
Parameter | Acid Chloride Route | Direct Oxidation Route |
---|---|---|
Starting Material | 3-Chlorobenzoyl chloride | 3-Chlorobenzoic acid |
Reaction Temperature | 15–25°C | 15°C |
Catalyst | H₂SO₄ | Benzyl triphenylphosphine chloride |
Solvent | Dioxane | Chloroform |
Yield | 92% | 85–90% |
Byproducts | Sulfuric acid salts | Manganese dioxide |
Scalability | Industrial | Laboratory-scale |
The acid chloride route remains dominant in industrial settings due to higher yields and established infrastructure. However, the PTC method offers greener chemistry by eliminating toxic solvent use and enabling catalyst recycling .
Properties
CAS No. |
5106-05-8 |
---|---|
Molecular Formula |
C7H5ClO3 |
Molecular Weight |
172.56 g/mol |
IUPAC Name |
2-chlorobenzenecarboperoxoic acid |
InChI |
InChI=1S/C7H5ClO3/c8-6-4-2-1-3-5(6)7(9)11-10/h1-4,10H |
InChI Key |
ULQQGOGMQRGFFR-UHFFFAOYSA-N |
Canonical SMILES |
C1=CC=C(C(=C1)C(=O)OO)Cl |
Origin of Product |
United States |
Disclaimer and Information on In-Vitro Research Products
Please be aware that all articles and product information presented on BenchChem are intended solely for informational purposes. The products available for purchase on BenchChem are specifically designed for in-vitro studies, which are conducted outside of living organisms. In-vitro studies, derived from the Latin term "in glass," involve experiments performed in controlled laboratory settings using cells or tissues. It is important to note that these products are not categorized as medicines or drugs, and they have not received approval from the FDA for the prevention, treatment, or cure of any medical condition, ailment, or disease. We must emphasize that any form of bodily introduction of these products into humans or animals is strictly prohibited by law. It is essential to adhere to these guidelines to ensure compliance with legal and ethical standards in research and experimentation.